Enantioselective Conversion of Racemic Felodipine to S(-)-Felodipine by Aspergillus niger and Lipase AP6 Enzyme ()
1. Introduction
Chirality is a fundamental property of biological systems and drug efficacy [1] . Interactions of drugs with receptors, enzymes or binding sites have long been known to be stereoselective. The two enantiomers of drugs often act differently with each other in bioenvironment and the enantiomers can exhibit different pharmacokinetic and pharmacodynamic properties [2] . The differences in pharmacodynamic and pharmacokinetic properties of enantiomer are related to the differences in affinity or intrinsic activity at receptor sites. The pharmacological activity may reside only in one enantiomer, while the other may be inactive or have desirable or undesirable activity [3] .
Production of the chiral molecule is one of the rapidly progressing fields of modern science. Importance of this field is growing continuously due to various therapeutic benefits of single enantiomer over racemate [4] . Besides the chemical methods, biocatalytic methods were also proved to be effective towards the generation of single active enantiomer from its racemate and the use of biocatalysts had been growing rapidly for the past few years [2] . Microorganisms have been shown the capacity for performing a wide range of metabolic biotransformations such as enantiomeric inversion and biocatalytic deracemization processes leading to enantiomerically pure compounds [5] [6] .
Felodipine belongs to the dihydropyridine class of calcium channel blocker indicated for the treatment of hypertension and stable angina pectoris. It possesses a chiral centre at the 4th position in the 1,4-dihydropyridine nucleus and exists as two enantiomeric forms [7] . It acts primarily on vascular smooth muscle cells by stabilizing voltage-gated L-type calcium channels in their inactive conformation. By inhibiting the influx of calcium in smooth muscle cells, Felodipine prevents calcium-dependent mycolyte contraction and vasoconstriction. S(−)-Felodipine was proved to be 3 times more potent than R(+)-Felodipine in an in vitro assay for coronary vasoconstriction. An in vivo study with spontaneously hypertensive rats showed that S(−)-Felodipine was 3 times more potent in lowering blood pressure [8] .
In view of this, it is important to use single active enantiomer rather than a racemate and hence in the present work the enantioselective conversion of racemic Felodipine to its active enantiomer by using the microbial cultures and enzyme was studied. To date, there are no reports available for biocatalytic transformation of Felodipine into its active S(−)-Felodipine. The microorganisms selected for the biotransformation study include Bacillus subtilis [9] , Escherichia coli [10] , Pseudomonas putida [11] , Sphingomonas paucimobilis [12] , Rhodococcus erythropolis [13] , Streptomyces halstedii [14] , Aspergillus niger [15] , Geotricum candidum [16] , Rhizopus oryzae [17] , Candida parapsilosis [18] , Cunninghamella elegans [19] , Cunninghamella blakesleeana [20] and enzyme triacylglycerol lipase from Aspergillus niger (Lipase AP6) [21] .
2. Materials
2.1. Chemicals
Racemic Felodipine was a gift sample from Aurobindo Pharmaceuticals Pvt. Ltd., Hyderabad (India). The enantiomers R(+)-Felodipine and S(−)-Felodipine were separated by Diacel Chiral Technologies (India) Pvt. Ltd., Hyderabad (India). Suitable growth media, triacylglycerol lipase from A. niger and all other chemicals were purchased from Hi-Media Laboratories Pvt. Ltd., Mumbai, India. The HPLC grade solvents were purchased from Sigma Aldrich Chemicals Pvt. Ltd., Mumbai (India). Analytical grade solvents purchased from Finar limited, Gujarat, India.
2.2. Microorganisms
The selected microbial cultures were purchased from Microbial Type Culture Collection and Gene Bank (MTCC), Chandigarh or National Collection of Industrial Microorganisms (NCIM), Pune, India. The cultures were revived, subcultured and stored in refrigerator at 4˚C. The bacterial and fungal cultures were maintained on suitable growth media.
2.3. Instrumentation
Incubation was done in refrigerated shaker incubator of model Innova 4230, New Brunswick Scientific Co., Inc. (NJ, USA). Sample analysis was done by using a chiral column (Lux Cellulose-4; 250 × 4.6 mm; 5 µ particle size) purchased from Phenomenex (USA) and an Ultra Fast Liquid Chromatograph of Shimadzu (Kyoto, Japan) equipped with binary pump (LC 20AD), UV/Visible detector (LC 20A) and Rheodyne injector port. Lab solutions software was used for the HPLC analysis.
3. Experimental Methods
3.1. Culture Procedure
In regular biotransformation experiments, a two stage fermentation protocol was followed. A loopful of culture from freshly grown agar slant was inoculated into 25 mL of sterile liquid growth medium and the flask was incubated at specific growth conditions (Table 1), at 120 rpm. After incubation this culture was considered as first stage culture and 500 µl of this culture inoculum was added to freshly prepared sterile liquid growth medium (25 mL) and was incubated at specific growth conditions at 120 rpm. After incubation, these second stage cultures were employed for the biotransformation studies. Fungal cultures were supplemented with 0.02% triton × 100 in order to get good dispersion of fungi in the media [22] .
3.2. Enantioselective Resolution of Felodipine Using Whole-Cell Microorganisms
The racemic Felodipine (2.5 mg/mL in methanol) was added to the second stage suspension cultures of all experimental organisms (25 mL culture in 100 mL capacity conical flask). Prior to addition, the drug solution was filter sterilized using sterile syringe driven PVDF hydrophilic membrane filters (pore size―0.22 µm). The flasks were gently shaken immediately after the addition of drug for its even distribution. Each culture was studied in triplicate (n = 3) while running suitable controls. Culture controls consisted of culture blanks in which the organisms were grown under identical conditions and instead of substrate these were added with 100 µL of methanol. Drug
![]()
Table 1. Growth conditions for selected microbial cultures.
controls were composed of sterile medium to which same amount of drug was added and incubated without microorganisms. The incubation was continued under similar conditions for 20 days and the samples were collected at the time intervals of 3rd, 5th, 10th, 15th, 20th day, extracted and analyzed by chiral HPLC [23] .
3.3. Enantioselective Resolution of Felodipine Using Immobilized Microorganisms
The entrapment method was adopted for immobilization of whole-cell microorganism of Pawar et al. (2012) [24] . The cells, which were previously washed with dipotassium hydrogen phosphate saline solution, were mixed with 3% sodium alginate solution with continuous stirring. The resulting suspension was added to an aqueous solution of calcium chloride (50 mM) in a drop wise manner. Calcium alginate beads were allowed to stand for 1h for hardening. The hardened beads were washed with distilled water and used for the biotransformation.
The organisms which were responded to whole-cell biotransformation were selected for immobilization method. Maximum enantioselective conversion of Felodipine was found in 10th day samples and hence in the immobilization study incubation was carried up to 10 days. The study was carried with suitable controls. Immobilized cells without drug substrate were considered as culture controls and drug in buffer solution without immobilized cells was considered as drug control. Both of these were maintained in phosphate buffer solution (0.5% K2HPO4 and 0.5% NaCl). All the culture flasks, except culture controls, were added with 2.5 mg/mL of the drug in methanol and were incubated in refrigerator incubator at 120 rpm for 10 days. Triplicate (n = 3) of the study was adopted.
3.4. Extraction and Sample Preparation of Felodipine
The samples from the culture flasks were collected at specified time intervals, and three volumes of ethyl acetate was added and vortexed for 1 min [25] . All the organic layers of ethyl acetate were combined and evaporated under vacuum and dried. The dried extracts were reconstituted with 1 ml of HPLC methanol and filtered with sterile syringe filter, and used for further analysis.
3.5. Enantioselective Resolution of Felodipine Using Free Enzyme
The reaction mixture was composed of n-hexane and isopropanol (6:4) along with racemic Felodipine (2.5 mg/mL) in methanol. The reaction was started by adding lipase (10 mg/mL) to the reaction mixture [26] and was incubated at 120 rpm, at 25˚C for 36 h. Samples were collected at intervals of 4th, 8th, 12th, 16th, 20th, 24th, 28th, 32nd, 36th h and evaporated at room temperature. The residues were dissolved in methanol (HPLC grade), filtered through membrane filter (pore size―0.22 µm) and analyzed by chiral HPLC.
3.6. Enantioselective Resolution of Felodipine Using Immobilized Enzyme
Enzyme immobilization was done by reported method [24] with few modifications. Briefly, enzyme (10 g/L) was suspended in sterile phosphate buffer (pH 6.8) and was mixed with equal volume of sodium alginate solution (3% w/v). Using a syringe, this suspension was extruded into calcium chloride solution (0.2 M) from a height of 15 cm to yield calcium alginate beads (2 mm) and kept aside (1 h) for hardening. Then they were washed thrice with distilled water and employed for the study by adding them into reaction mixture along with racemic Felodipine (2.5 mg/mL methanol). Reaction mixture with enzyme-alginate beads and without drug was considered as blank, while the drug control consisted of reaction mixture with the drug and without beads. All the test and controls were incubated at 25˚C at 120 rpm for 36 h, samples were collected (4th, 8th, 12th, 16th, 20th, 24th, 28th, 32nd, 36th h) and evaporated at room temperature. The residues were dissolved in methanol (HPLC grade), filtered through membrane filter (pore size―0.22 µm) and analyzed by chiral HPLC.
3.7. Chromatographic Analysis
The samples collected were analyzed by previously reported chiral HPLC method of Xu et al. [27] . The chromatographic conditions were shown in Table 2.
Enantiomeric ratio (E) is the parameter characteristic of the enantioselectivity of a particular biocatalyst and is independent of substrate concentration. It can be calculated by the formula [28] ;

where ‘eep’ is the enantiomeric excess of the product.
Enantiomeric excess (ee %) and extent of conversion (C %) were calculated by using the following formulae [29] [30] .
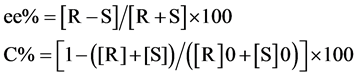
where R, S are the peak areas of the individual enantiomers and R0, S0 are the values of initial peak areas of the enantiomers.
4. Results and Discussion
Racemic Felodipine was analysed by the reported chiral HPLC method and the chromatogram was shown in Figure 1. Retention time of S(−)- and R(+)-Felodipine were found to be 14.16 and 20.22 min, respectively. Among the microbial cultures employed in the whole-cell catalyzed bioconversion study, six microbial cultures A. niger, S. paucimobilis, C. elegans, E. coli, P. putida and C. blakesleeana were able to catalyze the re-
![]()
Table 2. Chromatographic conditions.
![]()
Figure 1. Chromatogram of racemic Felodipine (1 µg/mL).
action with excess of the S(−)-Felodipine with the conversion rate ranges in between 25 to 55%. The enantiomeric purity of the drug was assessed by calculating the enantiomeric excess (ee%), conversion yield (C%) and enantiomeric ratio (E) and were shown in Table 3. The enantioselective conversion with the whole-cell cultures gradually increased upto 10th day of incubation and the maximum enantiomeric excess was obtained with 10th day samples. Among the whole-cell cultures catalysed studies, A. niger has given good enantiomeric excess while the remaining cultures yielded moderate enantiomeric excesses. Though all these cultures responded for the moderate to good enantioselective conversion, their enantioselectivity (enantiomeric ratio E) was found to be worst to poor.
The biocatalyst enantioselectivity can be improved by immobilization [32] . Hence all these cultures were immobilized. The reactions catalysed by immobilized cultures have yielded good enantiomeric purity of the drug. This may be due to immobilization which improved the enantioselectivity of the microbial cultures. E value of the reaction catalysed by A.niger was improved from poor to excellent after immobilization. Hence, the enantiomeric purity of the reaction was very good with ee% of 98.27% while the E value of the reaction catalysed by S. paucimobilis was improved from poor to good and hence yielded good enantiomeric purity after immobilization. The E values of reactions catalysed by C. elegans, E. coli and P. putida were improved from worst to moderate and hence resulted in enhanced enantiomeric excess after immobilization. E value of the reaction catalysed by C. blaksleana was improved from worst to poor and the enantiomeric excess was also enhanced after immobilization. Overall, immobilization of microbial cultures has leads to enhanced enantioselectivity of these cultures which resulted in improved enantiomeric purity of the drug when compared to the reaction catalysed by whole-cell cultures. The C% also enhanced after immobilization. The present results are in accordance with that of Ribeiro et al. (2014) where he has reported that the immobilized cells of Kluyveromyces marxianus resulted in enantioselective
![]()
Table 3. Enantiomeric purity of Felodipine after incubation with the whole-cell as well as immobilized-cell cultures for 10 days.
ee (%)―Enantiomeric excess, (C%)―Conversion yield, E―Enantiomeric ratio; *E value is considered as worst (1 - 5), poor (5 - 10), moderate (10 - 20), good (20 - 100) and excellent (>100) [31] .
reduction of benzyl acetoacetate to benzyl (S)-3-hydroxybutanoate with high enantiomeric excess (76%) and conversion rate (99%) than the free cells which resulted in low enantiomeric excess and conversion values (68% and 80% respectively) [33] . The results were also in line with Tamalampudi et al. (2007) and according to them (RS)-1- phenylethanol was enantioselectively transesterified to (R)-1-phenylethyl acetate by using immobilized recombinant Aspergillus oryzae expressing lipase-encoding gene from Candida antarctica, which resulted with an enantiomeric excess (ee) of >99%. The enantiomeric purity of (R)-1-phenylethyl acetate was found to be high when compared to that of the reaction catalyzed by whole-cell cultures [34] . The chromatograms of Felodipine after incubating with whole-cell and immobilized A. niger and S. paucimobilis cultures were shown in Figure 2(a) and Figure 2(b) and Figure 3(a) and Figure 3(b), respectively.
Among all reactions catalysed by immobilized cultures highest enantiomeric excess was resulted by immobilized A. niger and S. paucimobilis. This may be due to the presence of lipase enzyme is reported to be present in A. niger [35] . Lipase AP6 (Triacylglycerol lipase from A. niger) has employed as biocatalyst for the enantioselective conversion of racemic Felodipine. Enantiomeric purity of the drug after incubation with free and immobilized enzyme was shown in Table 4. The enantioselectivity of the
![]()
Figure 2. Chromatogram of Felodipine after incubation with (a) Whole-cell A. niger culture; (b) Immobilized A. niger culture.
![]()
Figure 3. Chromatogram of Felodipine after incubation with (a) Whole-cell S. paucimobilis culture; (b) Immobilized S. paucimobilis culture.
![]()
Table 4. Enantiomeric purity of Felodipine after incubation with free- and immobilized enzyme for 24 h.
*E value is considered as worst (1 - 5), poor (5 - 10), moderate (10 - 20), good (20 - 100) and excellent (>100) [31] .
free enzyme was found to be moderate and was resulted in good enantiomeric excess of the Felodipine. However the enantiomeric purity of drug was not so excellent and hence it can be improved by enhancing the enantioselectivity of the enzyme. Immobilization will improve the enantioselectivity of the biocatalyst [36] . E value (enantioselectivity) of the reaction catalysed by the enzyme was improved from moderate to excellent after immobilization and hence the enantiomeric excess was found to be excellent (99.21%). The conversion yield of the drug was also enhanced by reaction catalysed by enzyme after immobilization.
These results were in agreement with the results obtained by Alnoch et al. (2015) and Lee et al. (2011). They conclude that enzyme immobilization improved enantioselectivity and further leads to increased enantiomeric purity. According to the Alnoch et al. (2015) immobilized Lip G9 enzyme showed excellent enantiomeic ratio (E > 200) with high enantiomeric excess (ee% > 95) and conversion rate (49%) than the free enzyme [37] . Similarly Lee et al. (2011) reported the enantioselectivity enhancement by the use of immobilized Burkhoderia cepacia lipase is about 2 - 11 fold compared to that by the free enzyme [36]. On the whole, immobilization of the biocatalyst has improved their enantioselectivity and hence resulted in good to excellent enantiomeric purity of the drug. Chromatograms of Felodipine after incubation with free and immobilized enzyme for 24 h were depicted in Figure 4(a) and Figure 4(b), respectively.
5. Conclusion
In conclusion, immobilized lipase AP6 enzyme was found to be suitable biocatalyst for
![]()
Figure 4. Chromatogram of Felodipine after incubation with (a) Free-enzyme; (b) Immobilized- enzyme.
the enantioselective conversion of racemic Felodipine with excellent enantiomeric excess, followed by immobilized A. niger and S. paucimobilis cultures.
Acknowledgements
Authors are thankful for the financial assistance given by Science and Engineering Research Board (SR/SO/HS/0087/2010 dated 27/02/2012), Department of Science and Technology, New Delhi, India. Authors are also thankful to Aurobindo Pharmaceuticals Pvt Ltd, Hyderabad (India) for the kind gift of racemic Felodipine.