Novel Hydralazine Schiff Base Derivatives and Their Antimicrobial, Antioxidant and Antiplasmodial Properties ()
1. Introduction
Health problems have been a source of sadness and pain to humanity and research in the domain of health has afforded a great variety of therapeutics to fight against a wide range of diseases and ailments. Despite this effort and many others, some drugs have become ineffective as the micro organism develops resistance when the usage is recurrent, reason why illnesses like malaria, bacterial and yeast infections, gastrointestinal disorders, diarrhoea, dysentery, cholera, as well as chronic degenerative diseases like cancer, continue to inflict suffering in developing countries, Africa and the world. There is therefore urgent need to discover new drugs.
Condensation between primary amines and carbonyl compounds affords Schiff bases. The imine (-C=N-) functional group is a versatile pharmacophore for the design and development of biologically active leads. Schiff bases constitute an important class of organic compounds that show a wide variety of applications in many fields including the pharmaceutical and medicinal fields because of the wide range of biological activities they portray including anti-inflammatory [1] [2] [3] [4], antimicrobial [5] [6], antitubercular [7], antioxidant [8], anthelmintic [9], analgesic [2] [3] [4] [10] and anticonvulsant [11] activities among others. Apart from their pharmaceutical and medicinal importance, Schiff bases are widely used as catalysts, dyes, stabilizers in polymer formulations, pigments and corrosion inhibitory agents [12].
The growing resistance of infectious microorganisms to therapeutics is a serious concern and represents a health threat in both developed and developing world [13]. Introduction of new classes of antimicrobial drugs is desperately needed to hamper the tendency of drugs to quickly develop drug-resistant pathogens [13]. Therefore, drug discovery efforts will facilitate the identification of novel antimicrobial compounds and new drug combinations will facilitate the development of better dosing regimens and novel strategies to manage drug resistance and prevent the dissemination of resistant bacteria [14]. In Cameroon, infectious diseases such as malaria and bacterial infections are amongst the most commonly notified diseases and largest cause of mortality [15]. Malaria continues to be a major cause of childhood mortality and was responsible for an estimated 303,000 (165,000 - 450,000) deaths in children under 5 years old in 2015. The majority is caused by the Plasmodium falciparum infections. However, the 60% reduction observed in mortality since 2000 is heavily associated to the widespread deployment of effective control measures such as Artemisinin-based Combination Therapies (ACTs). The recent emergence and spread of Plasmodium falciparum parasites resistant to ACTs, worsened by the spread of insecticide resistance hindered the elimination of malaria [16] [17] [18].
An antioxidant is any molecule that delays, prevents or removes oxidative damage to target cells. Oxidative damage or oxidative stress produces free radicals which are dangerous to the body. Reactive oxygen species (ROS) is a term which encompasses all highly reactive molecules including free radicals like hydroxyl radical, superoxide anion radical, hydrogen peroxides, singlet oxygen, nitric acid radical and various peroxides [19]. When oxidative forces exceed the antioxidant systems, the cell is subjected to oxidative stress [19]. Oxidative stress is among the major causative factors in induction of many chronic and degenerative diseases including atherosclerosis, ischemic heart disease, aging, diabetes mellitus, cancer, immunosuppression, neurodegenerative diseases, male infertility, infectious diseases and others [20]. Therefore, development of new antimalarial, antibacterial and antioxidant chemotypes is an urgent priority. Our objective in this study is to synthesize new hydralazine-derived Schiff bases and to evaluate them for their antiplasmodial, antibacterial and antioxidant activity in vitro.
2. Experimental
2.1. Materials
Hydralazine hydrochloride, 4-acetylmorpholine, 2-hydroxybenzaldehyde and other solvents were purchased from commercial sources and used without further purification. All chemicals used were of reagent grade. Methanol was used as solvent all through the synthesis. Glass apparatus with standard interchangeable joint were used after being washed with concentrated sulphuric acid, distilled water and methanol. All the synthesis was carried out in a 250 mL round-bottomed flask fitted with a quick Liebig condenser where it involved refluxing. All reactions were performed on a hot plate equipped with a magnetic stirrer. Elemental analysis was performed on a VARIO EL (Heraeus) analyzer. IR spectra were obtained from a Perkin-Elmer spectrum 100-FT-IR spectrometer. 1H-NMR spectra were obtained on a Varian unity plus 400 MHz instrument. 13C-NMR spectra were recorded on a Bruker AV 100 MHz instrument.
2.2. Synthesis of 3-[1-(2-(Phthalazin-1-yl)Hydrazono)Ethyl)- 1,3-Oxazinane (PHEO)
The ligand was prepared by the condensation reaction between hydralazine hydrochloride and 4-Acetylmorpholine. (3 mmol, 0.6 g) of hydralazine hydrochloride and sodium acetate (buffering agent) (3 mmol, 0.4 g) was dissolved in 20 mL of ethanol, and (3 mmol, 0.4 g) of 4-Acetylmorpholine was also measured. The two solutions were transferred into a 250 mL round bottom flask and the mixture was refluxed for three hours while stirring at a temperature of 70˚C and allowed to cool overnight. The yellow precipitate obtained (PHEO) was separated by filtration, washed with ethanol and allowed to air dry giving a yield of 50%. The filtrate was sealed using paraffin in a vial and kept for further crystalization by slow evaporation to improve on the yield. The synthetic route for PHEO is shown in Scheme 1.
IR (KBr, cm−1): ν 3862, 3501, 1271, 1664, 1611, 970. 1H NMR (DMSO-d6, 500 MHz): 8.85 (s, 1H, N-H), 8.10 (dd, 1H, C-H of benzene ring), 2.45 (s, 3H, H-8’), 2.29 (t, 4H, H-3’, H-4’), 2.60 (t, 4H, H-6’, H-7’) ppm. 13C NMR (DMSO-d6, 125 MHz): 151.6 (C-1’), 135.9 (C-1), 134.2 (C-4) ppm.
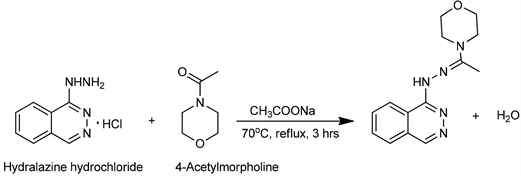
Scheme 1. Synthesis of PHEO.
2.3. Synthesis of 2-[(2-(Phthalazin-1-yl)Hydrazono)Methyl]Phenol (PHMP)
A (1.0 mmol, 0.201 g) of hydralazine hydrochloride and sodium acetate (buffering agent) (1.0 mmol, 0.137 g) was dissolved in 15 mL of ethanol and mixed with 0.104 mL of 2-hydroxybenzaldehyde. The solution was transferred into a 100 mL round bottom flask and then refluxed for three hours while stirring at a temperature of about 70˚C to 75˚C and allowed to cool overnight. The yellow precipitate (PHMP) was separated by filtration, washed with ethanol and allowed to air dry (yield = 76.05%). The filtrate was sealed using paraffin in a vial and kept for further crystalization by slow evaporation to improve on the yield. Scheme 2 shows the synthetic route for PHMP.
IR (KBr, cm−1): ν 3400, 3100.1, 1267.9, 1607.4, 960.9. 1H NMR (DMSO-d6, 500 MHz): 12.11 (s, 1H, OH), 10.30 (s, 1H, N-H), 8.69 (s, 1H, H-1’), 6.90 and 8.33 (benzene hydrogens of the hydralazine moiety) ppm. 13C NMR (DMSO-d6, 125 MHz): 157.0 (C-1), 154.0 (C-3’), 147.0 (C-1’) ppm.
2.4. Organisms and Growth Conditions
Microorganisms were obtained from the culture collections of the Antimicrobial and biocontrol Agents Unit at the Department of Biochemistry, University of Yaounde 1, Cameroon. Organisms were as follows: Streptococcus pneumoniae (Klein) Chester, ATCC49619, Staphylococcus aureus BAA 917, Staphylococcus aureus subsp. aureus Rosenbach, ATCC 43300, Staphylococcus aureus NR45003, Staphylococcus aureus NR46003, Staphylococcus aureus CP7625, Shigella flexineri NR518, Salmonella enterica subsp. enterica serovar AnatumNR4294, Salmonella enterica subsp. enterica serovar Muenchen NR4311, Salmonella enterica subsp. enterica, strain A36 is serovar TyphimuriumNR-13555, Pseudomonas aeruginosa NMC592, Klebsiella pneumoniae subsp. pneumoniae (Schroeter) Trevisan ATCC 13883, Klebsiella pneumoniae subsp. pneumoniae (Schroeter) Trevisan ATCC 700603, Klebsiella pneumonae NR41916, Escherishia coli ATCC25922, Escherishia coli ATCC35218, Enterococcus feacalis ATCC51219, Staphylococcus aureus NR46374, and Hemophyllus influenza ATCC49247. Organisms were maintained on Muller Hinton agar (MHA) (Oxoid). at 37˚C. Inocula were prepared by diluting overnight cultures in saline to 0.5 McFarland, approximately 108 cfu·ml−1. These suspensions were further diluted with medium as required.
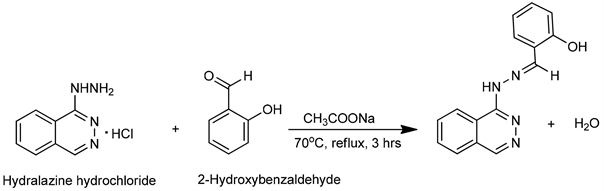
Scheme 2. Synthesis of PHMP.
Drug susceptibility Testing Procedure
Stock solution preparation
Stock solution of each sample was prepared at 100 mg/mL in DMSO.
2.5. Antimicrobial Assays
For the estimation of the antimicrobial activities of the plant extracts, a broth dilution method was employed for minimum inhibitory contraction (MIC) following the Clinical and Laboratory Standards Institute (CLSI) guidelines M07-A10. Each compound was tested in duplicate for MIC determination. More specifically, 50 µL of Mueller Hinton broth (MHB) were introduced in a 96-well microplate respectively. 50 µL of compound were added to wells of the first line. A serial twofold dilution was made by transferring 50 µL of the mixture of the first wells to the next one up to the last, final concentrations varying from 250 to 7.81 µg/mL. Then, 50 µL of an inoculum of 1 × 106 cells/mL were introduced in all the wells except those of the sterility control. The final concentration of DMSO was <1%. Each plate also contained a positive control, Amoxicillin, a negative control and a blank. Plates were incubated during 24 hours. The lowest concentration of compound that inhibited the visible growth of a microorganism was defined as minimum inhibitory concentration (MIC).
2.6. Antioxidant Assay
2.6.1. Organ Homogenates
A 2.5 month old male Wistar albino rat of 150 g was obtained from the Biochemistry animal house of the Faculty of Science of the University of Bamenda. They were handled according to ethical guidelines of the Cameroon National Veterinary Laboratory and were given food and water ad libitum. After sacrifice, the liver and testes were excised out, weighed and used to prepare 20% (W/V) homogenates using phosphate buffer (pH 7.4, 50 mM).
2.6.2. Chemicals
Ascorbic acid and Thiobarbituric, trichloroacetic acids were gotten from Griffin and George (Wembly Middlesex, England). Methanol was purchased from LobaChemiePvt. Ltd. 107, Woodehouse (Mumbai, India). Other chemicals were of high quality grade.
2.6.3. Evaluation of the Antioxidant Reducing Power
The antioxidant reducing power of the synthesized compounds PHEO and PHMP was evaluated using the method as described by Kamtekar and collaborators in 2014 [21]. The standard ascorbic acid or synthesized compounds (50, 100, 150, 200, 250 and 300 µg/mL) was introduced in the tube, then 0.4 mL of phosphate buffer (pH 6.6, 0.2 M) and 0.4 mL of 1% KFe(CN)6 were successively added. After homogenization, the mixture was incubated at 50˚C for 20 min, then cooled, and centrifuged (3500 rpm, 10 min, 25˚C). To 1 mL of supernatant was added 1 mL of 10% TCA, 1mL of distilled water and 0.2 mL of 0.1% FeCl3. After homogenization, the absorbance was measured at 593 nm using a UV-Vis spectrophotometer.
2.6.4. Inhibition of Lipid Peroxidation
A volume (0.8 mL) of phosphate buffer (pH 7.4, 50 mM) and 0.1 mL of liver or testis homogenate were added to standard ascorbic acid or synthesized compounds, PHEO and PHMP (50, 100, 150, 200, 250 and 300 µg/mL), followed by 0.1 mL of Fe2(SO4)2. After homogenization, the mixture was incubated at 37˚C for 15 min, then 1 mL of 20% TCA and 1 mL of 0.67% TBA, were added. The mixture was incubated at 90˚C for 10 min, cooled and centrifuged (3000 rpm, 15 min, 4˚C). The supernatant was collected and optical density read at 530 nm [22] and percentage inhibition (%I) of the standard and the synthesized compounds, PHEO and PHMP computed.
2.6.5. Catalase Activity
Ascorbic acid or the synthesized compounds, PHEO and PHMP (50, 100, 150, 200, 250 and 300 µg/mL) were introduced into test tubes containing 0.4 mL of 9% H2O2 and 2.3 mL of phosphate buffer (pH 7.2, 0.1 M) and 0.4 mL of the liver or testis homogenate were added. The absorbance was recorded at 240 nm using a UV-Vis spectrophotometer at 30, 60 and 90 seconds. Catalase activity was expressed as IU/mg protein [23].
2.6.6. Statistical Analyses
For ferric reducing power and lipid peroxidation, the fifty percent efficient (EC50) and inhibitory (IC50) concentration of the tested compound were determined. Differences between treatments were assessed by one factor ANOVA followed by the Student-Newman-Keulstest, and P values less than 0.05 were considered statistically significant. All analyses were performed using Graphpad Instat software Version 3.0.
2.7. Antiplasmodial Assay
SYBR Green I Based Fluorescence Assay
Drug sensitivity assay was carried out in 96-well microtitration plates using SYBR green I based fluorescence assay. Sorbitol-synchronized ring stage parasites, Plasmodium falciparum Dd2 (haematocrit: 3%, parasitaemia: 0.5%, 90 μl) under normal culture conditions were incubated in the presence of prediluted extracts and reference drug. The final concentration in the test plate was range from 0.781 to 100 μg/mL for extract and 0.0078 to 1 µM for artemisinin and chloroquine, in the total volume of 100 µL of culture. After 72 h of incubation, SYBR Green I was added and the fluorescence was measured using a Fluoroskan Ascent multi-well plate reader with excitation and emission at 485 and 538 nm, respectively. The fluorescence counts were plotted against the logarithm of sample concentration and the 50% inhibitory concentration (IC50) was determined by analysis of dose–response curves using GraphPad Prism 5. Experiments were done in duplicate. Cut off point for antiplasmodial activity of synthesized compounds is as follows: Highly active (IC50 ≤ 5 μg/mL), promisingly active (5 < IC50 ≤ 10 μg/mL), good activity 10 < IC50 ≤ 20 μg/mL, moderately active (20 < IC50 ≤ 40 μg/mL), marginally active (40 < IC50 ≤ 70 μg/mL), poorly active (70 < IC50 ≤ 100 μg/mL) [24].
3. Results and Discussion
The condensation reaction between hydralazine hydrochloride and 4-acetylmorpholine afforded PHEO (Figure 1) and that between hydralazine hydrochloride and 2-hydroxybenzaldehyde afforded PHMP (Figure 2).
3.1. Infrared Spectra
The IR spectrum of the PHMP (Figure 3) recorded in the 4000 - 400 cm−1 region displayed strong bands at 3400 cm−1 and 3100.1 cm−1 corresponding to the O-H and the N-H stretching vibrations respectively [25]. A sharp band was seen at
1267.9 cm−1 corresponding to the C-O stretch. Other strong bands were observed at 1607.4 cm−1 corresponding to the C=N stretch [26] and at 960.9 cm−1 attributed to the N-N stretch. This brought in to evidence the presence of these functional groups in PHMP.
The IR spectrum of the PHEO (Figure 4) recorded in the 4000 - 400 cm−1 region showed a band at 3862 cm−1 for the residual H2O molecule [25]. Also, sharp bands at 3501 cm−1 and 1664 cm−1 corresponding to the N-H stretch and the azomethine C=N respectively. Other sharp bands were observed at 1271 cm−1 and 970 cm−1 attributed to the C-O stretch and N-N stretch respectively. The band observed at 1611 cm−1 was that of the C=N stretch [26]. The very intense band at 1611 cm−1 is attributed to the C=C vibration [25].
3.2. 1H NMR Spectra
The proton NMR spectrum of PHMP (Figure 5) was recorded in deuterated DMSO as the solvent. The signal at 12.11 ppm (1H, s) is attributed to the O-H while that at 10.30 ppm (1H, s) is attributed to the N-H and is highly deshielded because of its tautomeric nature. A signal observed at 8.69 ppm (1H, s) was attributed to the methyne (-N=C-H) proton at carbon 1’. The group of signals between 6.90 ppm and 8.33 ppm has been attributed to the benzene protons of the hydralazine moiety. Figure 5 shows the 1H NMR spectrum of the PHMP.
The proton NMR spectrum of PHEO (Figure 6) was recorded in deuterated methanol (CD3OD) as the solvent. The signal at 8.85 ppm (1H, s) is attributed to the N-H of the hydralazine; deshielded because of its tautomeric nature, while that at 8.10 ppm (1H, dd) is attributed to the C-H of the benzene ring. The signal at 2.45 ppm (3H, s) is attributed to the methyl group while those at 2.29 ppm (4H, t) and 2.60 ppm (4H, t) are attributed to the two groups of methylene protons of the morpholine moiety. Figure 6 shows the 1H NMR spectrum of the PHEO.
The 13C NMR spectrum of PHMP (Figure 7) was recorded in deuterated DMSO as the solvent. It displayed 15 signals between 116.0 ppm and 157.0 ppm. The signals at 157.0, 154.0 and 147.0 were respectively attributed to carbons 1, 3’ and 1’.
The 13C NMR spectrum of PHEO (Figure 8) was recorded in deuterated DMSO as the solvent. The signals at 151.6, 135.9 and 134.2 were respectively assigned to carbons 1’, 1 and 4.
3.3. Anti-Plasmodial Activity
PHEO and PHMP were tested for anti-plasmodial activity using the SYBR green I based fluorescence assay and the results are presented in Table 1. PHEO inhibited the growth of the malaria parasites with a percentage inhibition of 78.55%, lower than the 80.41% obtained with the standard treatment of malaria, Artemisinin. Likewise, PHMP inhibited the growth of the malaria parasites with a percentage inhibition of 78.06%, lower than the 80.41% obtained with the standard treatment of malaria, Artemisinin. Considering that their percentage inhibitions in the preliminary assay were less than 80%, PHEO and PHMP were not considered for the Dose-Response studies.
3.4. Antimicrobial Activity
Antibacterial activities of PHEO and PHMP are recorded in Table 2. PHEO showed low activity only on one bacterial strain with MIC value of 250 µg/ml against Hemophyllus influenza ATCC49247. Antimicrobial activity of a compound is very interesting if the MIC is below 10 µg/ml, interesting or moderate if 10 < MIC £ 100 µg/ml and low if MIC > 100 µg/ml [15] [27]. Based on above-mentioned criteria, PHEO had low antimicrobial activity. PHMP was effective (interesting activity) against 4 bacterial strains out of 19 with MIC of 31.25 - 250 µg/ml against Hemophyllus influenza ATCC49247; Staphylococcus aureus NR46374; Staphylococcus aureus BAA 917 and Streptococcus pneumoniae
![]()
Table 1. Antiplasmodial activity of synthetic compounds.
ART = artemisinine; CQ = chloroquine; N.D. = Not determined.
![]()
Table 2. Antibacterial activities of PHEO and PHMP.
(Klein) Chester ATCC49619. However, the results achieved can serve as starting point for lead optimisation in order to generate more active lead compounds that can be developed into new antibacterial drugs.
3.5. Antioxidant Activity
3.5.1. Ferric Reducing Powder and Lipid Peroxidation
The effects of different compounds and vitamin C on reduction of ferric ions and peroxidation of lipids are presented in Table 3. In general PHEO and PHMP showed higher reducing activity on ferric ions than the reference compound vitamin C. On lipid peroxidation, PHEO showed higher inhibition (P < 0.05) while PHMP showed lower inhibition (P < 0.05) compared to vitamin C.
3.5.2. Catalase Activity
The catalase activity in the presence of PHEO, PHMP and Vitamin C is depicted in Figure 9. Catalase activity showed concentration dependent effect in the presence of all compound. In general Vit C presented higher stimulatory effect on the enzyme activity than PHEO and PHMP. PHMP showed a lower effect on catalase activity.
4. Conclusions
PHEO and PHMP were synthesized from hydralazine hydrochloride and characterized by spectroscopic methods.
PHEO showed low anti-microbial activity only on one bacterial strain with MIC value of 250 µg/ml against Hemophyllus influenza ATCC49247. PHMP showed interesting activity against 4 bacterial strains out of 19 with MIC of 31.25 - 250 µg/ml against Hemophyllus influenza ATCC49247; Staphylococcus aureus NR46374; Staphylococcus aureus BAA 917 and Streptococcus pneumoniae (Klein) Chester ATCC49619.
For antioxidant activity, PHEO and PHMP showed higher reducing activity on ferric ions than the reference compound Vit C and on lipid peroxidation,
![]()
Table 3. Effects of compounds and Vit C on reduction of ferric ions and peroxidation of lipids.
*P < 0.05 compared to the reference Vit C, Student-Newman-Keuls test.
![]()
Figure 9. Effects of compounds and Vitamin C on catalase activity.
PHEO and PHMP showed higher inhibition than Vit C. Catalase activity showed concentration dependent effect in the presence of all compounds. In general, Vit C presented higher stimulatory effect on the enzyme activity than PHEO and PHMP. PHMP showed a lower effect on catalase activity.
PHEO and PHMP inhibited the growth of the malaria parasites with percentage inhibitions of 78.55% and 78.06% respectively; lower than the 80.41% obtained with the standard treatment of malaria, Artemisinin. Considering that their percentage inhibitions in the preliminary assay were less than 80%, PHEO and PHMP were not considered for the Dose-Response studies.
The anti-microbial, anti-oxidant and anti-plasmodial activities of these compounds are reported for the first time and the results indicate that these novel ligands can be exploited as anti-microbial and anti-oxidant agents.
Acknowledgements
We are grateful to the Department of Chemistry, University of Buea for providing the necessary support to perform the experiments.