Design of Low-Voltage, Low-Power FGMOS Based Voltage Buffer, Analog Inverter and Winner-Take-All Analog Signal Processing Circuits ()
Received 1 December 2015; accepted 10 January 2016; published 13 January 2016

1. Introduction
Current trends in consumer electronics strive to achieve low-voltage and low-power circuit designs of voltage buffer, analog inverter and WTA having utility in portable electronics. Floating Gate MOS technology is a low voltage design technique with its attractive features such as reduced circuit complexity and simplified signal processing chain of a design. It is capable of shifting the signal levels and incorporating tunable mechanisms due to its programmable threshold voltage. It operates normally below the operational limits of supply voltage levels for a particular technology and thus consumes less power than the minimum power required for a CMOS circuit of same technology without compromising on device performance [1] . Applications of FGMOS transistors in neural networks [2] , voltage-controlled resistors [3] , electronic programming [4] , current mirrors [5] , digital-to- analog and analog-to-digital converters [6] , multipliers [7] , squarers [8] , operational transconductance amplifier [9] , etc. have been reported. Exclusive features such as flexibility, controllability and tenability of FGMOS that yield better results in terms of power supply voltage motivated us to propose FGMOS based Voltage Buffer, Analog Inverter and WTA.
Voltage buffer circuit implemented using CMOS technology has been reported in literature extensively. However, some FGMOS based voltage buffers have also been reported, to refer some of them, K. Moolpho and J. Ngarmnil [10] have proposed low voltage FGMOS based class AB buffer error amplifier comprising of three main building blocks in which one of the blocks is the two input gate additive inverter using FGMOS devices and Gupta M. et al. [11] have presented improved FGMOS based low power voltage follower circuit. In this paper, we have implemented a new FGMOS based voltage buffer circuit which dissipates less power.
Implementation of FGMOS inverter to generate the nonlinear functions has been proposed by Trejo-Guerra, R. et al. [12] . Performance related properties such as power, delay, power-delay product (PDP), and energy-delay product (EDP) of FGMOS inverter operating in subthreshold region are investigated in [13] . Differential Floating Gate analog inverter has been reported in [14] . Here, we propose an FGMOS based differential difference voltage buffer (FGDDVB) circuit based on the well-established and versatile Differential Difference Amplifier (DDA) circuit topology.
WTA networks are an important class of circuits widely used in neural networks and pattern-recognition systems [15] . Not much work has been published on FGMOS based WTA circuit. A novel FGMOS based Winner-Take-All (WTA) circuit with reduced power dissipation is presented.
Thus, in summary, this paper introduces FGMOS based analog signal processing circuits―voltage buffer, analog inverter and winner-take-all. Simulated results are provided to demonstrate reduced power dissipation and an increase in the input range due to the use of floating gate transistors.
The paper is organized as follows. The operation of FGMOS transistor is described in Section 2. Proposed FGMOS based voltage buffer, analog inverter and WTA circuits are presented in Section 3. Simulation results are discussed in Section 4 to verify theoretical results. Finally, conclusions are drawn in the last section.
2. Basics of FGMOS Transistor
FGMOS transistor has multiple inputs whose gate is subject to capacitive couplings between itself and input terminals. Threshold voltage of the transistor is tuned by capacitor values and applied bias voltage. Figure 1 shows equivalent representation of FGMOS transistor [1] .
The input signals (
) are applied at floating gates
of FGMOS transistor, respectively. The voltage on floating gate VFG is given as [1] 
![]()
Figure 1. Equivalent representation of FGMOS.
where Ci is the set of capacitors associated with effective inputs and the floating gate. Total floating gate capa-
citance is given by
where CFGD, CFGS and CFGB are overlap capacitances of
floating gate with drain, source and bulk, respectively, VD is the drain voltage, VS is the source voltage, VB is the bulk voltage, and QFG is the residual charge trapped in the oxide-silicon interface during fabrication process. By the justification suggested in [8] , neglecting residual charge, QFG and assuming
, CFGB [1] , the drain current of FGMOS transistor in saturation region can be expressed as
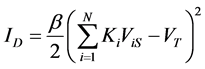
where ki = Ci/CT, β is the transconductance, VT is the threshold voltage.
3. Circuit Descriptions
3.1. Proposed FGMOS Voltage Buffer
Analog voltage buffers play a significant role in mixed signal designs where they are used for signal monitoring and for driving large capacitive loads [16] . Voltage buffer with floating gate transistors has been implemented with modifications being made to the circuit of voltage buffer implemented using CMOS whose structure is similar to differential difference amplifier [17] , published in [18] .
The proposed FGMOS circuit is obtained by replacing the matched differential stage (M1 and M2), by two input floating gate transistors. The configuration is similar to DDA realization, but the first and second inputs are removed; hence the third input and output become a voltage follower building block. The complete FGMOS voltage buffer is shown in Figure 2. PMOS transistors operate in saturation region described by the following equation:
![]()
where
, VTP is threshold voltage, μP is surface mobility, COX is channel oxide capacitance, W is channel width and L is channel length of the transistor. M1 and M2 operate in saturation region and their drain currents can hence be written as:


With current mirrors M3 and M4 perfectly matched,
.

Cancelling the common terms gives,

![]()
If Vin2 is zero, then V0 = Vin1, i.e., the output follows the input, thus the circuit operates as a voltage buffer.
3.2. Proposed FGMOS Analog Inverter
Differential difference voltage buffer (DDVB) [19] basically consists of two voltage buffers which invert the input to the first buffer from the input to the second buffer, hence acts as an analog inverter. CMOS implementation of DDVB has been presented in [17] .
Proposed FGMOS analog inverter is obtained by replacing MOSFETs M1, M2, M3 and M4 in DDVB circuit [19] by two input floating gate transistors which results in circuit of Figure 3. All p-type and n-type MOSFETs as well as floating gate transistors in the proposed circuit operate in saturation region.
3.3. Proposed FGMOS Based WTA Circuit
The proposed n-input FGMOS WTA maximum circuit as indicated in Figure 4 is drawn on similar lines as CMOS WTA max circuit published in [18] . All MOSFETs and floating gate transistors operate in strong inversion saturation region with VGS > VT. The operating principle remains the same.
4. Simulation Results
The conventional and proposed FGMOS based analog signal processing circuits are simulated using SPICE (Pspice version 16.5) in CMOS 180 nm technology. Rail-to-rail input range is assumed and a supply voltage of ±1.25 V is taken for simulating both circuits. The aspect ratios of the transistors of the conventional circuits and proposed circuits are listed in Table 1. The performance parameters of the proposed circuits and their corresponding conventional circuits are compared in Table 2. To overcome DC convergence error during simulation because of the floating gate (FG) of FGMOS, model suggested in [1] has been used in all proposed FGMOS based circuits. In this model, very high value resistors (RG) are connected in between the FG node and a set of voltage sources.
DC response of CMOS voltage buffer and FGMOS based voltage buffer is shown in Figure 5. Proposed FGMOS voltage buffer was implemented with two inputs to floating gate MOSFET M1. Output was observed at one of the inputs to M2, with input to the MOSFET being grounded. It can be seen from the DC analysis that the operating (linearity) range increases in case of FGMOS voltage buffer compared to CMOS voltage buffer. While it can be observed from simulation that the proposed FGMOS based voltage buffer justifies its operation in the entire supply voltage range, i.e., from −1.25 V to 1.25 V, CMOS based voltage buffer operates as a buffer in the voltage range from −1.25 V to 1 V. This is evident due to the fact that the effective input signal at the floating gate is scaled down by equivalent capacitance ratio (Ci/CT), which results into a lower distortion and hence a larger input range is achieved. Frequency response of conventional and proposed voltage buffer is shown in Figure 6. DC response for CMOS analog inverter and the proposed FGDDVB are shown in Figure 7. Simulation results reveal that power dissipation reduces drastically for the proposed FGDDVB. The linearity range has increased for the proposed FGDDVB (0 to 1 V) compared to the conventional CMOS based analog inverter (0 to 0.9 V). Frequency response of conventional and proposed analog inverter are shown in Figure 8. It
![]()
Table 2. Comparison of conventional and proposed circuits.
![]()
Figure 5. DC response of conventional CMOS and proposed FGMOS based voltage buffer.
![]()
Figure 6. AC response of conventional CMOS and proposed FGMOS based voltage buffer.
![]()
Figure 7. DC response of conventional CMOS and proposed FGMOS based DDVB.
![]()
Figure 8. AC response of conventional CMOS and proposed FGMOS based DDVB.
can also be seen that the proposed configuration operates at lower supply voltage compared to the conventional DDVB. DC response and frequency response of CMOS WTA and the proposed FGMOS based WTA are shown in Figure 9 and Figure 10 respectively. From the simulation results it can again be observed that power dissipa-
![]()
Figure 9. (a) DC response of conventional CMOS WTA; (b) DC response of proposed FGMOS WTA.
![]()
Figure 10. AC response of conventional CMOS and proposed FGMOS based WTA.
tion is reduced for proposed WTA circuit. In general, for all the proposed FGMOS based analog signal processing circuits, power dissipation is reduced compared to the conventional circuits proposed earlier.
5. Conclusion
In this paper, novel FGMOS based analog signal processing circuits―voltage buffer, analog inverter and winner-take-all circuits are presented. All proposed circuits operate at ±1.25 V. A larger operating range is achieved for FGMOS based voltage buffer and FGMOS based analog inverter compared to their corresponding conventional circuits, as desired. Power dissipation is reduced in all the proposed FGMOS based circuits, thus making these circuits useful in low-voltage and low-power applications. The proposed circuits are intended to find applications in low voltage, low power consumer electronics.
Acknowledgements
The work was carried out at Netaji Subhas Institute of Technology, New Delhi (University of Delhi) as a partial fulfillment of engineering degree.
NOTES
![]()
*Corresponding author.