Effect of propofol on local field potential in rat prefrontal cortex during working memory task ()
1. INTRODUCTION
1.1. Propofol
Propofol (2,6-diisopropylphenol) is an intravenous anesthetic that has been gaining use for induction and maintenance of anesthesia in clinical practice during the last 10 years. With the use of propofol there are some research reports about memory dysfunction caused by propofol anesthesia especially in elderly patients [1-3]. It remains unclear, however, whether these cognitive or memory changes are due to the effects of propofol.
1.2. Working Memory
Working memory is the ability to actively hold information in the mind needed to do complex tasks such as reasoning, comprehension and learning [4]. Working memory tasks are those that require the goal-oriented active monitoring or manipulation of information or behaviors in the face of interfering processes and distractions. The cognitive processes involved include the executive and attention control of short-term memory which provide for the interim integration, processing, disposal, and retrieval of information.
1.3. Memory Mechanism of Neuron Cell Assemble
Hebb’s cell assembly theory states that “Neurons do not individually represent memories but a large group of simultaneously active neurons account for any concept or idea in the human brain. Central to his theory is the idea of cell assemblies, which are formed as a result of the proposed learning process” [5]. So we research effects of propofol on working memory through the electric activities of neuron assembles.
1.4. Prefrontal Cortex
Behavioral studies have shown that damage in the prefrontal cortex (PFC) impairs performance in working memory tasks across various mammalian species, indicating that the PFC plays an essential role in working memory [6]. Physiological studies suggest that working memory is mediated by continuous activities of PFC neurons [7,8]. These studies have led to the current prevailing view that persistent neuronal activity in the PFC plays an essential role in mediating working memory [9-11].
1.5. Local Field Potential
The local field potential (LFP) is composed of low-frequency extracellular voltage fluctuations that are thought to reflect synaptic potentials and other slow electrical signals such as spike afterpotentials and voltage-dependent membrane oscillations [12,13]. Neurophysiologists have used the LFP with increasing frequency in recent years to link neural activity to perception and cognition, including sensory stimuli coding, perceptual binding, attention, and working memory [14-22].
In the present study, we aimed to determine effects of propofol anesthesia on working memory. To test this, the propofol infusion continued at a rate of 0.9 mg·kg−1·min−1 via a tail vein catheter for two hours. And then we simultaneously recorded LFPs from the PFC during working memory test of adult rats, using chronically implanted multielectrode arrays [23]. At last we tested the effect of propofol in rats in both LFPs and behavior tests.
2. MATERIALS AND METHODS
2.1. Subjects and Housing Conditions
A total of 20 male Sprague-Dawley rats (approximately 3 months), purchased from Institute of Radiation Medicine Chinese Academy of Medical Sciences, were housed in standard shoebox cages, had free access to drinking water, and were kept under a 12-h light-dark cycle. The rats’ care and surgical procedures were in accordance with the National Institutes of Health Guide for the Care and Use of Laboratory Animals (1997) and with the Tianjin Medical University guidelines for the use and care of laboratory animals in research.
2.2. Y-Maze Training Procedure
Rats were food deprived to 85% of their free-feeding body weight. Behavioral training was conducted in a Ymaze (Figure 1). Both the start arm (75 cm long) and the two arms forming the Y (both 75 cm long and diverging at a 120˚ angle from the stem arm) were 5 cm in width. Each animal was given 3 days of 30 min pre-training in order to train them to run reliably down the stem of the maze and to find peanut tablets in the food wells in both arms.

Figure 1. Y type maze used for rats behavior training and testing.
Following pre-training, the training began. At the start of training, which consisted of two stages, two peanut tablets were placed in each food well and a translucent plastic block was placed at the neck of one arm. As a consequence, on each “sample run” the rat would enter the open arm where it was allowed to eat the food at the end of the arm. The rat was then picked up and confined at the start stem for a delay of 10 s, during which the translucent plastic block was removed. The door at the start arm was then opened and the animal allowed a free choice between the two arms of the Y-maze. On this “choice run”, the animal was considered “right” if it had entered the arm not previously entered on the “sample run” and would then be allowed to eat the food reward before being returned to the start stem. If the rat returned to the arm visited on the “sample run”, it was recorded “incorrect” and confined to that arm for 5 s before being returned to the breed box. The training procedure was not ended until each rat’s the correct rate was over 80%.
2.3. Surgical Procedure
The surgical procedure has already been described in detail [24]. All SD rats were implanted with custom-built 16-wire-S-isonel-coated tungsten microwire electrode arrays (30 μm diameter wires with (600 - 800 kΩ) impedance, arranged in a 2 × 8 grid with 200 μm spacing between wires) in the PFC under propofol (0.5 mg·kg–1·min–1) anesthesia. The following coordinates (in millimeters) relative to Bregma were used to center the arrays: PFCx (2.5 - 4.5 mm anterior to bragma and 0.2 - 1.0 mm lateral to midline, 2.5 - 3 mm deep from cortical surface).
2.4. LFPs Recording and Processing
Rats were allowed 7 days to recover from the surgery. We separated all the rats into two groups. Ten rats were injected propofol at a rate of 0.9 mg·kg–1·min–1 via a tail vein catheter for two hours; and the other ten rats, injected no propofol, were as the control.
After 12 hours, all the propofol group rats have recovered from anesthesia and moved as freely as the control ones. Two group rats were placed into the Y-maze to do the working memory test one by one. The test procedure was the same as the training procedure. Every rat completed continuous 10 tests in the morning and continuous 10 in the afternoon, with inter-test interval 2 min. The rats received 20 tests a day until the two rat groups’ correct rates had no differences.
At the same time, LFPs were recorded from the multielectrodes arrays and continuously sampled at 2 kHz by using Cerebus 128 Channel Data Acquisition System (Cyberkinetics, Inc., US). The LFP signal was band-pass filtered between 0.3 - 500 Hz to remove low-frequency direct current fluctuations and reduce high-frequency noise. Then 50 Hz power line noise was removed by applying a band pass-filter to the original data, and subtracting the resulting filtered signal from the original data.
Estimate instantaneous phase by Hilbert transform: For an arbitrary signal x(t), the analytic signal is a complex function of time defined as Eq.1.
(1)
where the function
is the Hilbert transform of
,
instantaneous phase.
Calculate cross-correlation coherence: if
and
are the instantaneous phase corresponded to two sequences of LFPs, the cross-correlation value is calculated as Eq.2.
(2)
where
is time delay,
is cross-correlation value [25,26].
Cross-correlation coherence is used to estimate the degree of synchronization between two signals in the frequency domain. Cross-correlation values are normalized in the [0,1] interval.
The zero coherence means completely desynchronized, while unit coherence means in perfect synchrony between the two signals.
In this study, we compute the cross-correlation coherences of 16-channel LFPs in 4 s during rats’ working memory test. They are obtained by 50 ms sliding window and 25% overlapping. As the second channel has medium average neural firing rate of neurons, it is used as the reference channel to do the coherence with other channels.
2.5. Data Analyses
Data from working memory test and cross-correlation coefficient between the two LFPs were tested using repeated measures ANOVA. A limit for significance was P < 0.05. All data are given as means ± SEM.
3. RESULTS
Results are based on data from 20 rats and 400 experimental sessions.
3.1. Working Memory Y-Maze Test
For the 3 days of test, where each test was separated by an inter-trial interval of 2 min and comprised a sample and choice run, an analysis carried out on the total correct choices revealed a significant group difference [F(1,18) = 14.7, P < 0.01] in the first day and [F(1,18) = 7.3, P < 0.05] in the second day as the propofol group made fewer correct choices than the control group; while there is no difference [F(1,18) = 2.6, P > 0.05] in the third day (Figure 2).
But the propofol group’s performance improved over the test days [F(2,27) = 5.6, P < 0.05], whereas the control group performance stayed stable [F(2,27) = 2.4, P > 0.05].
3.2. Cross-Correlation Coherence between LFPs during Working Memory Test
We measured the LFP signals 400 times from prefrontal cortex in 20 rats while they engaged in working memory task. Figure 3 shows raw data of the LFPs recorded from prefrontal cortex during a single test. Figures 3(a), (c) and (e) show raw data of LFPs recorded from one of the propofol rats in the first, second and third day respectively. Figure 3(b), (c) and (f) show raw data of LFPs recorded from one of the control rats in the first, second and third day respectively.
We calculated the average cross-correlation coherences of the LFPs across working memory task. Especially, we focused on cross-correlation coherences of LFPs around 2 s preand post-event, which was triggered by infrared in Y-maze.
Figure 4 depicts the average cross-correlation coherences of the LFPs preand postthe working memory event. No significant differences in the average crosscorrelation coherences of the LFPs of propofol group were found between the pre and post the working memory event in the first [F(1,398) = 1.5, P > 0.1] and second day [F(1,398) = 1.9, P > 0.1]. By contrast, the average cross-correlation coherences of propofol group increased significantly between the pre and post the working memory event in the third day [F(1,398) = 8.1, P < 0.01].
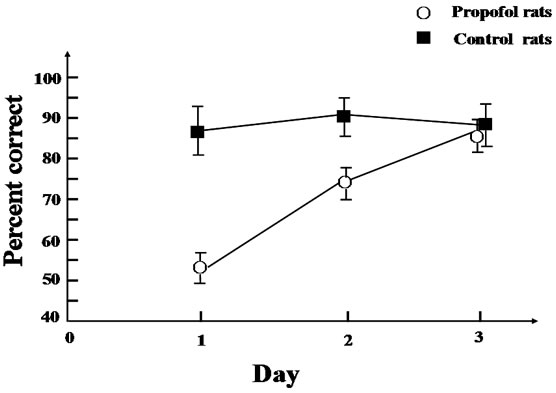
Figure 2. Reinforced alternation in the Y-maze. Mean correct choices rate (±SE) during the test sessions where each test comprised a sample run and a choice run and an inter-trial interval of 2 min (continuous 10 tests in the morning and continuous 10 in the afternoon one rat one day).
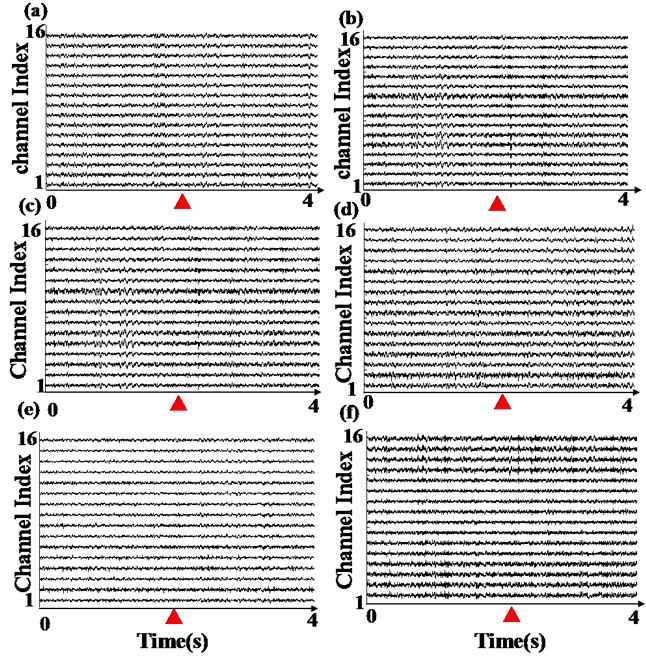
Figure 3. Example of LFP records. These data represent one test of each rat of two groups which contributed to the cross-correlation coherence estimates.▲ represent the tripping time by infrared in Y-maze. (a), (c) and (e) represent one of propofol rat’s LFP of first, second and third test day; (b), (d) and (f) represent one of control rat’s LFP of first, second and third test day.
(a)
(b)
(c)
Figure 4. The average cross-correlation coherences of the LFPs of two groups pre and post the working memory event. (a) Presents the first day; (b) Presents the second day; (c) Presents the third day. (*present P < 0.05, **present P < 0.01).
As to the control group, the average cross-correlation coherences increased significantly between the pre and post the working memory event every day (P < 0.01).
The results indicate that propofol impairs the rats’ working memory and cross-correlation coherence of LFPs in the prefrontal cortex. Our results suggest that Propofol can impair cross-correlation coherence of LFPs in the first two days, but not long time.
4. DISCUSSION
The purpose of this study was to evaluate the effects of propofol on working memory. From the result we can find out some common character: the propofol groups’ correct choice rate is fewer than the control group in the first and second day; LFPs of propofol group can not form significantly synchronization in the first and second day either. In the third day there is no difference between the propofol and control group in behavior choice, and LFPs of propofol group rats can form significantly synchronization as the control group rats do. So we discover that whether LFPs of prefrontal cortex form significantly synchronization is relative to the rats’ correct choice rate in the Y-maze, which can reflect function of rats’ working memory. Propofol anesthesia can interfere with LFPs of rats’ prefrontal cortex, and LFPs can not form meaningful synchronization when rats’ working memory is formed in the Y-maze. But the effect of propofol on LFPs is not unrecoverable. On the third day, the effect of propofol on LFPs is disappeared, and LFPs can form significantly synchronization, then rats can form working memory again.
This research demonstrates that working memory is unimpaired in young rats when testing begins 3 days after general anesthesia with propofol. It is coincident with other studies which demonstrate that psychomotor and memory function are impaired for only 24 h or less after surgery under propofol anesthesia [27-29]. Propofol inhibits synaptic transmission in the central nervous system primarily by enhancing GABAA receptor currents while having smaller effects on NMDA receptor/channels. NMDA receptor blockade produces long-lasting changes in hippocampal LTP and LTD [30]. So propofol only partially inhibits LTP and has no effect of LTD [31]. LTP and LTD represent electrophysiological correlates of use-dependent efficacy of the synapse and are used to study mechanisms of memory formation. These evidences can also proof propofol anesthesia decreases working memory function during short time.
We use LFPs to reflect the effect of propofol on working memory for a number of reasons. First, the temporal structure of the LFP has been shown to reflect sensory and motor-related signals that can be modulated by cognitive processes. The LFP therefore provides additional information to single neuron activity [32]. Second, the source of LFPs is largely from synaptic activity, Thus, LFPs represent the inputs and local processing. Third, the cortical LFP is easy to record, even over long periods of time. Finally, compared with EEG, local field potential signals have better time and spatial resolution.
The degree of correlation between two LFPs can be calculated by use of mutual correlation function. There will be huge calculation if we calculate cross-correlation value of two LFPs among all 16 channel LFPs. So in this paper we select the LFP channel in which neurons with the highest discharge frequency exist during the working memory event as the reference channel, and calculate cross-correlation value between other 15 LFPs with reference LFP using method described in Toshiyuki, H. et al. [33]. Huge calculation can be saved by use of “reference” LFP.
However, the design of this study has important limitation. Because cognitive impairment is common immediately after surgery and general anesthesia in elderly patients, the three month old rats are as the youth for people, not the old ones. So in the future we will do more research about effect of propofol on working memory of aged rats.
In total, the result indicates that in young rats memory is not persistently impaired by propofol and that the anesthetic state is neither necessary nor sufficient for development of postanesthetic memory impairment.
5. ACKNOWLEDGEMENTS
This work was supported by two grants from the Natural Science Foundation of China (Grant No. 61074131 and Grant No. 91132722).
NOTES