Clarithromycin Attenuates the Bronchial Epithelial Damage Induced by Mycoplasma pneumoniae Infection ()
1. Introduction
Mycoplasma pneumoniae is an atypical bacterium that causes acute respiratory infections, including pharyngitis, tracheobronchitis, and community-acquired pneumonia [1] -[3] . M. pneumoniae infection is initiated by attachment of the organism to ciliated cells, which is followed by ciliostasis, the alteration and loss of cilia, and destruction of the mucosal epithelial cells [4] -[6] . The cytoadherence ability of M. pneumoniae, which activates immune responses, is involved in the pathogenesis of M. pneumoniae infection [7] . In addition, we have also reported that interleukin (IL)-18 and IL-8 play important roles in the pathogenesis of M. pneumoniae infection according to disease severity in humans [8] [9] . These results have indicated that the pathogenesis of M. pneumoniae infection is mediated by host immune reactions.
Macrolide antimicrobials, such as clarithromycin, are first-line drugs of choice for the treatment of M. pneumoniae infections. Macrolides have also been hypothesized to have immunomodulatory activities that are independent of their known antimicrobial activities [10] -[12] .
Tagliabue et al. have reported that M. pneumoniae-infection-induced lung damage is attenuated by clarithromycin treatment in a dose-dependent manner in a murine model [13] .
In order to evaluate the morphology of the bronchial epithelial cell damage induced by M. pneumoniae infection and the therapeutic activities of clarithromycin in mouse model, here we observed bronchial tissue damage via immunostaining, scanning electron microscopy, and differential interference contrast (DIC) microscopy in a mouse infection model.
2. Materials and Methods
2.1. Organism and Growth Conditions
We used the M. pneumoniae FH (ATCC 15531) type strain. M. pneumoniae was cultured in a dish containing 5 mL of PPLO broth (with 1% tryptone, 0.5% glucose, 0.9% yeast extract, 1% TC yeastolate, and 17% newborn calf serum) at 37˚C for 5 days. After removing the supernatant, 200 μL of new PPLO broth was added to the dish. M. pneumoniae was collected from the bottom of the dish using a cell scraper. The concentration of this M. pneumoniae was about 108 CFU/mL.
2.2. Animals and Inoculation
Specific-pathogen-free female mice (BALB/cCrSlc; 10 weeks of age) were purchased from Japan SLC, Inc. (Hamamatsu, Japan). The mice were housed in filter-top cages for a week, to acclimatize them to the new environment. During the M. pneumoniae inoculation, the mice were anesthetized by administering sodium pentobarbital (75 mg/kg) intraperitoneally. The mice were administered 1 transnasal inoculation of 25 μL of 108 CFU/mL of M. pneumoniae. The mice were reared at 24˚C ± 2˚C in a 12 h - 12 h light-dark cycle and given free access to food and water. All of the mice received equal daily care.
2.3. Treatment Regimen
The mice were orally administered 20 mg/kg of clarithromycin (Taisho Pharmaceutical Co., Ltd., Tokyo, Japan) or a placebo once per day for 6 days, beginning the day after inoculation with M. pneumoniae. Clarithromycin was suspended in 5% gum arabic, and the same amount of 5% gum arabic was orally administered as a placebo. This infection experiment was repeated 3 times.
2.4. Sample Collection
The mice were sacrificed 6 days after the infection in order to obtain their lung tissue. The lung tissue was collected after anesthetization with sodium pentobarbital (75 mg/kg, intraperitoneal). The lung tissue was used in the following experiment, after being washed with Hanks’ balanced salt solution.
2.5. Immunohistochemistry
The collected lung tissue samples were fixed with 4% paraformaldehyde. The primary bronchus was resected and washed with phosphate-buffered saline (PBS) containing 0.05% Tween 20 (TPBS) and then blocked for 30 min with 1.5% horse serum that was diluted with TPBS. Next, the samples were processed at 37˚C for 90 min with a rabbit anti-M. pneumoniae polyclonal antibody (GeneTex, Inc., Irvine, CA, USA) that was diluted to 1:10 with TPBS and then washed with TPBS. Next, the samples were incubated at 37˚C for 30 min with a biotinylated goat anti-rabbit IgG polyclonal antibody (Vector Laboratories, Inc., Burlingame, CA, USA) that was diluted to 1:250 with TPBS. After washing with TPBS, the samples were incubated at room temperature for 30 min with ABC reagent (Vector Laboratories, Inc.) that was diluted to 1:100 with TPBS. After another washing with TPBS, the samples were processed at room temperature for 30 min with streptavidin and Alexa Fluor® 488 conjugate (Life Technologies Corporation, Grand Island, NY, USA) that was diluted to 1:500 with TPBS. After a final washing with TPBS, the primary bronchus were cut open lengthwise, placed on a slide with the mucosal surface up, and enclosed with a 22-mm square cover glass after adding 20 μL of ProLong Gold Antifade Reagent (Life Technologies Corporation). Digital photographs of the Alexa Fluor® 488 fluorescent sections were obtained using a LSM-700 laser scanning confocal microscope and the attached software (Carl Zeiss AG, Oberkochen, Germany).
2.6. Observation with a Scanning Electron Microscope
The collected lung tissue samples were fixed with 4% paraformaldehyde. The primary bronchus was resected, cut open lengthwise, and washed with PBS. Subsequently, the bronchial tissues were postfixed in 2% osmium tetroxide for 3 h in an ice bath. The specimens were then dehydrated in graded ethanol and dried to CO2 critical point dry. Dried specimens were coated with an osmium plasma ion coater and submitted for scanning electron microscope observation (JEM-6320F, JEOL Ltd., Tokyo, Japan).
2.7. Observation with an Optical Microscope
The primary bronchus was resected from the collected lung tissue samples and cut open lengthwise in Hanks’ balanced salt solution and was washed well. The bronchial samples were placed mucosal surface-up on a glass base dish (Aashi Glass Co., Ltd., Tokyo, Japan), and 1 mL of Hanks’ solution was added. The respiratory tract submucosal samples were visualized via DIC microscopy performed using a Nikon Eclipse Ti-E inverted microscope (Nikon Corporation, Tokyo, Japan) and photographed with a Canon EOS5D digital camera (Canon Inc., Tokyo, Japan).
3. Results
3.1. Location of M. pneumoniae-Labeled Fluorescence
Six days after M. pneumoniae inoculation, the mice bronchi were resected, fixed in 4% paraformaldehyde, and stained with fluorescent anti-M. pneumoniae antibodies. The mucosal epithelium of the primary bronchus was observed using a laser scanning confocal microscope. M. pneumoniae-labeled fluorescence was not observed on the mucosal epithelium of mice that were not inoculated with M. pneumoniae (Figures 1(a)-(f)). M. pneumoniae-labeled fluorescence was found on the mucosal epithelium of the mice inoculated with M. pneumoniae (Figures 1(g)-(i)). Fluorescence was observed on the cilia of the epithelial cells, indicating that M. pneumoniae adhered to the cilia of the ciliated cells and infected the mucosal epithelium (Figures 1(j)-(l)). Decreased fluorescence was observed in the mice that were administered with clarithromycin (Figures 1(m)-(r)). M. pneumoniae-labeled fluorescence was attached to the cilia, suggesting that clarithromycin might help in the elimination of M. pneumoniae from the epithelial cells.
3.2. Damage to the Bronchial Mucosal Epithelium
The mice lungs were collected 6 days after inoculation with mycoplasma, and the surface of the mucosal epithelium of the primary bronchus was observed by scanning electron microscopy. While ciliated cells completely covered the bronchial surface of the mucosal epithelium in mice not inoculated with mycoplasma (Figure 2(a), Figure 2(b)), scattered ciliated cells were observed on the mucosal epithelium of the mice inoculated with mycoplasma (Figure 2(c), Figure 2(d)). In addition, the lengths of the cilia were shortened. Among them, cilia had fallen off some ciliated cells (Figure 2(d) arrows). In order to observe the mucosal fluids on the trachea, the mice lungs were collected, and the surface of the mucosal epithelium of the primary bronchus was observed using DIC without fixation. A number of fragmented cilia were observed in the mucosal fluids on the epithelium (Figure 2(g), Figure 2(h)). These fragmented cilia were not observed in the mucosal fluids on the epithelium of the mice that were not inoculated with M. pneumoniae (Figure 2(i)). Many ciliated cells were observed on the mucosal surface, the cilia length was maintained and almost no ciliated cells had lost cilia in the clarithromycin-

Figure 1. Laser scanning confocal microscopic images of mouse bronchus 6 days after Mycoplasma pneumoniae inoculation. Fluorescent staining with anti-M. pneumoniae antibodies were performed, and laser scanning confocal microscopy was used to observe the M. pneumoniae in the mucosal epithelium of the primary bronchus. Images a-f show the mucosal epithelium of a mouse not inoculated with M. pneumoniae; images g-l show the mucosal epithelium of a mouse inoculated with M. pneumoniae; and images m-r show the mucosal epithelium of a mouse consecutively administered with clarithromycin (20 mg/kg) once per day beginning the day after M. pneumoniae inoculation. M. pneumoniae adhered to cilia and infected the bronchus of the mouse inoculated with M. pneumoniae. Almost no mycoplasma was observed to adhere to the cilia on the mucosal epithelium of the mouse that was administered with clarithromycin. a, d, g, j, m, and p are fluorescent images; b, e, h, k, n, and q are differential interference contrast (DIC) images; and c, f, i, l, o, and r are merged fluorescent and DIC images. These images were obtained using an 63× oil objective with a 0.5 zoom factor (a-c, g-i, and m-o) and a 2.0 zoom factor (d-f, j-l, and p-r).
treated mice (Figure 2(e), Figure 2(f)), unlike that in case of the mice who were not administered clarithromycin.
3.3. Inflammatory Cells in the Bronchial Submucosal Tissue
Observations of the bronchial submucosal tissue with DIC microscopy showed numerous inflammatory cells that were thought to be neutrophils in the submucosal tissue of the mice inoculated with mycoplasma (Figure 3(a), Figure 3(c), Figure 3(e)). Figure 3(b), Figure 3(d), and Figure 3(f) are schematic views that show the inflammatory cells as white circles. The presence of many inflammatory cells in the submucosal tissue of the mice inoculated with mycoplasma was thought to be due to inflammation that was caused by mycoplasma, which caused the inflammatory cells to migrate to the tissue. Mice administered with clarithromycin beginning the day after M. pneumoniae inoculation exhibited less inflammatory cell migration than the mice that were not administered with clarithromycin.
4. Discussion
4.1. Mycoplasma pneumoniae Infection-Induced Damage and Inflammation of the Bronchial Mucosal Epithelium
The pathogenic mechanisms of the M. pneumoniae infection and the host response have not been well understood. In the present study, we observed morphological alterations of bronchial ciliated cells in a murine model of M. pneumoniae respiratory infection. We clearly demonstrated morphological alterations of bronchial mucosal epithelium, including the shortening and loss of cilia and a submucosal inflammatory response of inflammatory cell migration.
In terms of pathogenesis, M. pneumoniae is thought to principally act as an extracellular pathogen by attaching to the surface of the respiratory ciliated epithelium [3] . Hydrogen peroxide and superoxide radicals that are

Figure 2. Scanning electron microscopic images of mouse bronchus, 6 days after M. pneumoniae inoculation. The surface of the mucosal epithelium of the primary bronchus was observed. Images a and b show the mucosal epithelium of a mouse not inoculated with M. pneumoniae; images c and d show the mucosal epithelium of a mouse inoculated with M. pneumoniae; and images e and f show the mucosal epithelium of a mouse consecutively administered clarithromycin (20 mg/kg) once per day beginning the day after M. pneumoniae inoculation. The mucosal epithelial cells are colored blue, and the cilia are colored orange. Scattered ciliate cells with shortened cilia are seen on the mucosal epithelium of the mouse inoculated with M. pneumoniae. The arrows point to ciliate cells that have lost cilia. Ciliate cell density and cilia length were maintained on the mucosal epithelium of the mouse administered with clarithromycin. The mucosal epithelium of mouse inoculated with M. pneumonia, were visualized by DIC microscopy (g, h). Image h is a high magnification of part of the white square of image g. A number of fragmented cilia were observed in the mucosal fluids on the epithelium. Image i shows the mucosal epithelium of a mouse not inoculated with M. pneumoniae, that was visualized by DIC microscopy.The scanning electron microscopic images of mouse bronchus were obtained at a magnification of 1000× (a, c, and e) or 3000× (b, d, and f). The DIC images of the mucosal epithelium were obtained at an objective magnification of 40×.
synthesized by M. pneumoniae act in concert with endogenous toxic oxygen molecules that are generated by host cells to induce oxidative stress in the respiratory epithelium [14] . Recently, an ADP-ribosylating and vacuolating cyototoxin (designated CARDS toxin) of M. pneumoniae has been identified that may induce the epithelial injury that occurs with infection [15] . These pathogenic mechanisms of M. pneumoniae may lead to the deterioration of the cilia of respiratory epithelium, both structurally and functionally. The cells may lose their cilia entirely, ultimately resulting in the exfoliation of all or parts of the infected cells [5] [6] .
We have reported that IL-18 and IL-8 play important roles in the pathogenesis of M. pneumoniae infection according to disease severity [8] [9] . It can be reasonably speculated that IL-18 functions as a regulator for T helper type 1 cytokines and that IL-8 acts as a mediator for neutrophilic inflammation. In this study, we demonstrated morphological alterations of tracheal mucosa, including the shortening and loss of cilia (Figure 2(c), Figure 2(d)), and the infiltration of neutrophils in the submucosal tissue of the mice (Figure 3(a), Figure 3(c), Figure 3(e)).
4.2. Effects of Clarithromycin Treatment against Tracheal Damage Induced by Mycoplasma pneumoniae Infection
Clarithromycin treatment reduced the fluorescence on the epithelium of M. pneumoniae-inoculated-mice (Figure 1) andattenuated the damage of the ciliated cells (Figure 2). Furthermore, mice that were administered with
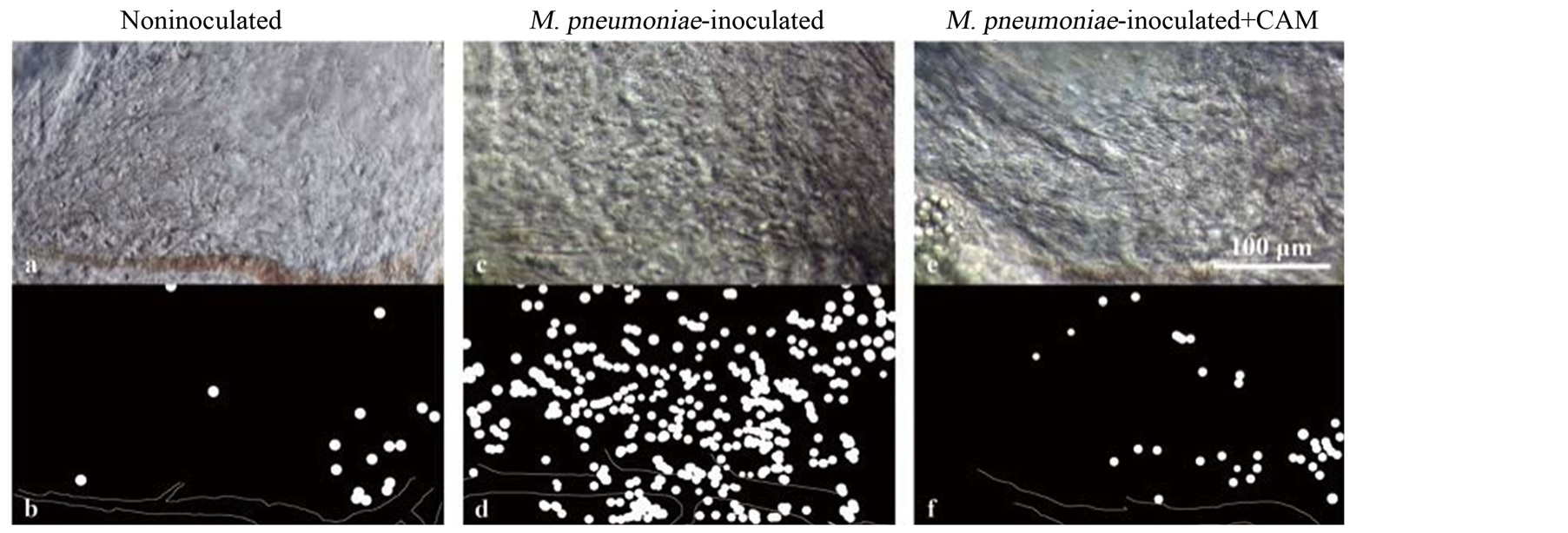
Figure 3. DIC images of the submucosal tissue of mouse primary bronchus, 6 days after M. pneumoniae inoculation. Image a shows the submucosal tissue of a mouse not inoculated with M. pneumoniae; image c shows the submucosal tissue of a mouse inoculated with M. pneumoniae; and image e shows the submucosal tissue of a mouse consecutively administered clarithromycin (20 mg/kg) once per day beginning the day after M. pneumoniae inoculation. Images b, d, and f are schematic views of a, c, and e, respectively, which show inflammatory cells as white circles and blood vessels as white lines. Numerous inflammatory cells can be seen to have migrated to the submucosal tissue of the mouse inoculated with M. pneumoniae. In the submucosal tissue of the mouse administered with clarithromycin, there were fewer inflammatory cells than in the submucosal tissue of the mouse not administered with clarithromycin. The DIC images of the submucosal tissue of the mouse primary bronchus were obtained at an objective magnification of 40×.
clarithromycin, beginning the day after M. pneumoniae inoculation, exhibited less inflammatory cell migration than the mice that were not administered with clarithromycin.
Tagliabue et al., have reported that clarithromycin (10, 25 and 75 mg/kg/day) resulted in a significantly reduced M. pneumonia quantitative culture, resulting in improvements in markers of disease severity and a significantly reduced lung histopathology score. In this study, we showed similar resultsof a morphological alteration with immunostaining and microscopic techniques in a mouse infection model. The elimination of mycoplasma by clarithromycin and its immunomodulatory effects were thought to have suppressed the migration of the inflammatory cells.
However, a limitation of this study was the concentration of clarithromycin. In this study, we used only one dose (20 mg/kg/day) of clarithromycin. A dose-response study is required for further analyzing the optimal dose of clarithromycin for treatment.
4.3. Possible Role of the Immunomodulating Activities of Clarithoromycin in the Mechanisms of the Therapeutic Activity of Mycoplasma pneumoniae Infection
Clarithromycin has been hypothesized to have immunomodulatory activities that are independent of their known antimicrobial activities, such as decreasing IL-8 production and neutrophilic inflammation [10] -[12] . Tamaoki et al., have also reported that macrolide antibiotics increase ciliary motility in rabbit airway epithelium [16] . These results indicate that the action of macrolides enhance the mucociliary clearance mechanisms and help the clearance of M. pneumoniae on the epithelial ciliated cells. Kurata et al., have shown that clarithromycin treatment decrease the number of macrolide-sensitive and resistant M. pneumoniae in the lungs in a mouse model [17] . In addition, they have indicated that the clarithromycin has adequate effects on M. pneumoniae infection by palliating the excessive inflammation that arises from the host immunoresponse.
These results indicate that, at least partly, the therapeutic effects of clarithromycin in this murine model were due to the immunomodulating activities of clarithromycin. Further clinical studies are needed to clarify the contribution and mechanisms of the immunomodulating activities of clarithromycin in treating acute respiratory tract infections, such as M. pneumoniae infection, in patients.
Ethical Standard
For the animal experiments, all of the procedures and animal care were conducted according to the Guidelines for Proper Conduct of Animal Experiments established by the Science Council of Japan in 2006.