1. Introduction
WC-Co cemented carbides are consist of large amounts of faceted carbide grains embedded in a ductile binder and due to their remarkable mechanical properties such as high hardness, high wear resistance and high temperature strength are one of the most important materials for cutting and mining tools [1,2]. Ultra fine/nano grains WC-Co have attracted wide interest, because of their superior hardness and fracture toughness [3]. It has shown that improvement of mechanical properties of cemented carbide alloys mainly relies on proper using of grain growth inhibitors and development of the appropriate microstructure and grain sizes through sintering process [2]. WC-Co hard materials can be densified by liquid phase sintering and mechanical properties of these materials depend on their composition and microstructure particularly the average grain size and size distribution of WC grains [4]. It is important to minimize the occurrence of discontinuous grain growth since the mechanical properties are adversely affected by a wide range of distribution of grain sizes. In WC-Co cemented carbide alloys, improvement of mechanical properties has been studied by addition of various grain growth inhibitors in the alloys. Among the various inhibitors studied, vanadium carbide and chromium carbide are the most effective grain growth inhibitors because of their high solubility in Co phase at lower temperature [5]. The mechanism of grain growth inhibition is based on the slowing down of solution/reprecipition reactions at WC-Co interfaces [6].
The work presented here was therefore stimulated by considering the possible advantages of co-addition of the above mentioned additives at the situation of this research. Here we report the effect of addition of amounts of Cr3C2 and Cr3C2-VC and various sintering temperatures in H2 atmosphere. These parameters effect on microstructure and mechanical properties of WC-%10wt Co alloys and obtain fine WC grains and improve hardness and fracture toughness.
2. Experimental
The chemical composition and average particle size of powders which were used in this study are shown in Table 1.
Small additions of Cr3C2 (0.3%, 0.6%, and 1% by weight) and VC (0.7% by weight) were added to the WC-%10wt Co powders prior to ball milling. Mechanical milling was carried out in a RETSCH PM400-MA type high energy ball mill for 10 hours and balls to powder weight ratio equal to 15 and selected rotation velocity of 200 rpm and 1:–3 rotation ratios of the sun wheel to grinding jar in milling to produce WC nano powders with particle size less than 50 nm [7].
After milling, powders were dried at an oven at 100°C for 24 hours in a rough vacuum. The nano powders were placed in a cylindrical steel mould with 10 mm diameter and were compacted at 200 MPa pressure. Then the compact samples were sintered 1370°C - 1450°C for 1 hour in high purity H2 atmosphere. The sintered samples were ground and polished with diamond paste. Microstructural study of the polished sample was carried out with Philips XL-30 scanning electron microscope. Average grain sizes of WC at the bulk WC-%10wt Co and the effects of Cr3C2 and VC grain growth inhibitors on microstructural variations also observed by back scattered electron SEM images.
Hardness and fracture toughness of samples were determined by Vickers hardness by 30 kg load and Palmqvist equation, respectively [8].
3. Results and Discussions
Figure 1 shows the hardness of samples as a function of sintering temperature. As can be seen from this figure, hardness of Cr3C2 containing samples are improved with increasing of sintering temperature.
The 1 wt% Cr3C2 containing sample has the lowest amount of hardness. This sample even has lower hardness than the samples without grain growth inhibitor additives. Cr3C2 as a translational carbide has a supersaturated point in liquid Co and probably with increasing of inhibitor content after this point. Excessive Cr3C2 inhibitor precipitates at WC/Co grain boundaries, deteriorates properties. With increasing sintering temperature up to 1410°C, hardness of most samples with grain growth inhibitors are increased (Figure 1). This related to increasing of fluidity of liquid cobalt phase, which are the filling voids in the samples.
However, with increase of sintering temperature above 1410°C, diffusion coefficient of carbon and tungsten atoms in cobalt phase increases and the hardness of alloy decreases (Hall-Petch relationship) [9]. Figure 2 displays the fracture toughness of sintered alloys as a function of sintering temperature. It shows that the fracture toughness of WC-Co-Cr3C2 samples improve with increasing of sintering temperature.
The observed increasing of fracture toughness at high temperatures can be possibly ascribed to liquid cobalt phase which fills the voids in the samples rarely i.e., improving of fracture toughness. The fracture toughness of VC containing samples is the lowest ones. Previous studies have reported the presence of VC as grain growth inhibitor produced brittle phases and so fracture toughness decreased [10].
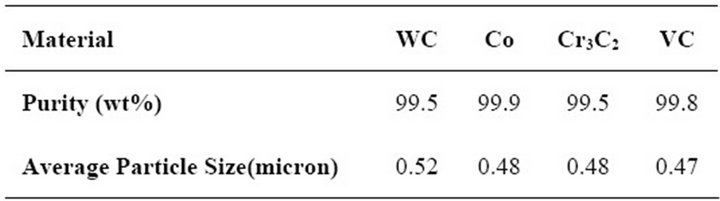
Table 1. Characteristics of initial powder.
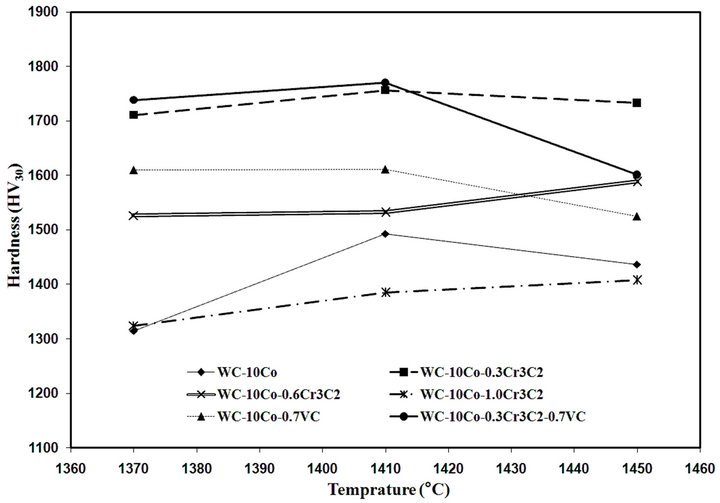
Figure 1. Hardness of the samples at the various sintering temperatures.

Figure 2. Fracture toughness of the samples at the various sintering temperatures.
As can be seen in Figure 2, the fracture toughness of WC-Co-Cr3C2 samples has decreased with increasing of sintering temperature. This is possibly related to the crystallization of brittle amorphous phase in the splat boundaries and precipitation of fine carbide. It is noteworthy that the fracture toughness decreases abruptly above the sintering temperature of 1410°C. Decreasing of fracture toughness is may be due to the presence of considerable amount of eta phase (Co6W6C) resulted in depletion of ductile Co [9]. Figures 3(a)-(f) demonstrates typical SEM micrographs of the samples have sintered at 1410°C. As can be seen from these figures, with addition of Cr3C2 and VC singly or simultaneously as grain growth inhibitors, the size of WC particles is mainly decrease.
Table 2 shows the average grain size of WC particles at the sintering temperature of 1410°C. It is observed that the average grain size of WC in WC-10%Co-0.3% Cr3C2-0.7%VC sample is about 0.45 micron. This is half of the samples without grain growth inhibitors.
The comparison of average grain size obtained for all the samples suggests that co-addition of VC and Cr3C2 is more effective to reduce the average grain sizes compared to those samples without one of the grain growth inhibitors. Anyway, Cr3C2 as a growth inhibitor reduces carbide solubility and causes a reduced growth rate of WC grains [11]. WC grain shape especially the truncation of the triangular prism is reported to depend on the potential of alloy. Co content is observed to change the elongation of WC grains. Additions of transition metal carbides also influence grain morphology. Cr3C2 additions generate partly rounded WC grains [1]. While VC induces sharp triangular grains, it should be mentioned that different diffusion rates can be anticipated for different atomic species which could vary in different ways as sintering progress. Previous studies have reported the dissolving of Cr3C2 carbide in liquid Co and reducing of the solubility of W and C atoms in liquid Co and will hence inhibit the rate of WC grain growth [5]. Also the availability of the grain growth inhibitors in WC-Co interface affects the interfacial free energy. For Cr3C2-VC containing samples, the observed sharp decrease of average grain size compared to other samples is due to both grain growth inhibitor and decrease of solubility of WC in Co phase and also these carbides act as a diffusion barrier and will hence inhibit WC grain growth.
4. Conclusions
1) Vanadium carbide and chromium carbide are the effective inhibitors against WC grain growth in liquid phase sintering. These carbides improve the mechanical properties of WC-10Co composite.
2) Addition of 0.3 wt% Cr3C2 in WC-10Co nanocomposite improves hardness and fracture toughness simulationsly.
3) With co-addition of 0.3 wt% Cr3C2 and 0.7 wt% VC, hardness of the samples increase to 1770 (HV30) and fracture toughness to 7 MPa
and average grain sizes decrease to 0.45 micron.

Table 2. Average grain size of WC at 1410°C sintering temperature.
4) The fracture toughness of WC-10Co-0.3Cr3C2 at 1410°C abruptly increases to 15.1 MPa
and average grain sizes decrease to 0.53 micron.
NOTES