Evaluation of the Microbiological Quality of Poultry Imported into Togo and the Antibiotic Resistance of Salmonella spp. Isolated ()
1. Introduction
In recent years, the agri-food industry, and in particular the livestock sector, has been rocked by a number of crises: food poisoning in the United States caused by salmonellosis and E. coli 015.H7, and the epidemic of bovine spongiform encephalopathy (BSE), avian influenza and influenza AH1N1 in Europe [1] . The main source of human infection is the ingestion of contaminated food, particularly raw or undercooked meat, mainly poultry [2] . Yet meat remains a highly prized foodstuff, thanks to its high nutritional value. It is rich in nutrients, particularly essential amino acids, making it an almost irreplaceable food. It is also easy to digest, which is at least part of the reason for the rapid development of the meat products industry and all the related commercial transactions [3] . The nutrients provided by meat, such as protein, are considered essential for human growth [4] . Of all animal sources, beef and chicken are the main sources of meat protein and are widely consumed in many countries. Meat is considered a potential medium for microbial growth due to its high protein content and high nutritional value [5] . In Togo, there is a major shortfall in the coverage of meat product needs, and per capita consumption of meat and offal per year is estimated at 7.5 kg, whereas the recommended standard is 12 kg per person per year. Over the last 5 years, this figure has risen by just 0.1 percentage points per year. As a result, the country imports around 30,000 head of cattle, 40,000 small ruminants, a million live poultry and almost 10,000 tons of meat a year to cover the needs of the population. Because imported chicken meat is cheaper than locally produced meat, broiler numbers on poultry farms have been falling steadily since 2003 [6] . As a result, food quality has become a major concern for consumers in many countries, including Togo [3] . It is therefore imperative to examine the microbiological quality of broiler meat in order to assess the risk it may pose to public health [7] . Several studies on pathogenic germs in meat have been carried out. However, they have not systematically taken into account the prevalence of germs, the frequency of meat of unacceptable hygienic quality, and the impact of the presence of these germs in the fight against antimicrobial resistance. Studies carried out in Africa report high prevalence of Escherichia coli and Salmonella in chickens [7] [8] . This high prevalence of pathogenic germs is compounded by very high levels of resistance to the antibiotics tested [9] . This study was conducted to estimate the frequency of unsatisfactory hygiene in imported poultry and to determine the antibiotic resistance profile of the Salmonella strains isolated.
2. Materials and Methods
2.1. Period, Study Area and Sample Collection
From 10 November 2020 to 03 June 2021, 285 samples of whole chicken (n = 5), chicken armor (n = 10), chicken forequarters (n = 35), chicken wings (n = 25), chicken thighs (n = 55), chicken drumsticks (n = 95), guinea fowl wings (n = 30) and sausages (n = 30) were collected from seven (7) cold stores in Lomé. The samples were transported directly to the laboratory for analysis in insulated coolers within 1 hour of sampling. The locations of the sampling sites are shown on the map in (Figure 1).
Source: QGIS Date: March 2023 Real: TOUGLO KOSSI
Figure 1. Map of Lomé (Préfecture du Golf) with the sites where sampling was done (A, B, C, D, E, F, G).
2.2. Microbiological Analyses
TAMF, Escherichia coli, Anaerobic Sulphito Reducing, coagulase-positive Staphylococci and Salmonella were tested and enumerated. Twenty-six grams (26 g) of meat were aseptically sampled and placed in a stomacher bag to which we added up to 260 mL of tap peptone water; then the stomacher was agitated for 2 to 3 minutes. This produces a 1:10 dilution of the product. After homogenizing the stock suspension in the Stomacher, dilutions from 10 to 10 were made from the stock solution at 10-1, each time taking 1 mL added to 9 mL of peptone water in a test tube.
TAMF was enumerated on Plat Count Agar (PCA) medium according to NF EN ISO 4833-1 (2013), Escherichia coli was enumerated according to NF ISO 16649-2 (2001) on Tryptone Bile X Glucuronide (TBX) medium and Staphylococcus aureus according to NF EN ISO 6888-1 (1999) on Baird Parker medium (supplemented with 1% potassium tellurite egg yolk). AnaerobicSulphitoReducing (ASR) bacteria were enumerated in accordance with NF ISO 15213 (2003) on Tryptone Sulfite Cyclo-serine (TSC) agar and Salmonella were detected on Xylose Lysine Desoxycholate (XLD) and Hecktoen agar media in accordance with NF EN ISO 6579-1 (2017).
The results were calculated and expressed using the following formula:
N = number of microorganisms; V = volume of inoculum; a = number of colonies in the first dilution; b = number of colonies in the second dilution; d1 = dilution rate; 0.1 = dilution factor.
The criteria for meat products (FCD, 2021) with M = 10 m were used to interpret the results according to the 3-class plan [10] .
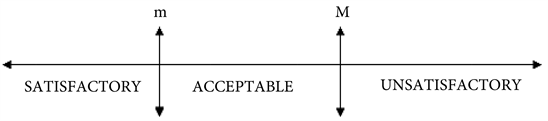
m: represents the threshold value and M represents the limit of acceptability.
Calculations of standard deviations (CFU/g ± standard deviation) in relation to average results were carried out.
2.3. Serological Identification and Antibiogram of Isolated Salmonella Strains
Isolated Salmonella strains were serologically identified by slide agglutination with anti-somatic O antigen sera to determine the group, followed by agglutination with flagellar H antigen sera to identify the serotype within the group according to the Kaufmann-White scheme. Antibiotic susceptibility testing was carried out by the agar disc diffusion method using Mueller Hinton agar (Kirby-Bauer).
Interpretation was carried out in accordance with CA-SFM 2023 [11] , using the reference strain Escherichia coli ATCC 25922 for quality control. The antibiotic discs (Liofilchem, Italy) used were as follows: Amoxicillin (20 μg), Amoxicillin + clavulanic acid (20/10 μg), Ticarcillin (75 μg), Ticarcillin + clavulanic acid (75/10 μg), Piperacillin (30 μg), Piperacillin + Tazobactam (30/6 μg) Cefalexin (30 μg), Cefoxitin (30 μg), Cefuroxime (30 μg), Ceftazidime (10 μg), Ceftriaxone (30 μg), Cefepime (30 μg), Aztreonam (30 μg), Imipenem (10 μg), Ertapeneme (10 μg), Amikacin (30 μg), Gentamicin (10 μg), Tobramycin (10 μg), Chloramphenicol (30 μg), Trimethoprim-sulfamethoxazole (25 μg), Ciprofloxacin (5 μg), Ofloxacin (5 μg), Nalidixic acid (30 μg), Levofloxacin (5 μg), Fosfomycin (200 μg), Nitrofurantoine (100 μg).
3. Results
3.1. Prevalence of germs isolated in the various samples
3.1.1. Prevalence of Total Aerobic Mesophilic Flora
TAMF was detected in 185/285 (71.71%) of the samples, with total contamination of guinea fowl wings, chicken wings and chicken armor. It should also be noted that all samples from chambers A, E and G were contaminated with TAMF (Table 1).
3.1.2. Prevalence of Escherichia coli
Escherichia coli was detected in 94/285 (32.98%) of the samples and chicken wings were the most contaminated with 30/30 (100%). Samples from cold rooms A, E and G were all contaminated (Table 2).
3.1.3. Prevalence of Anaerobic Sulphito Reducing
The samples were 42/285 (14.73%) contaminated with RSA and the chicken wings
![]()
Table 1. Total Aerobic Mesophilic Floracarriage rates in samples.
Legend: CUP: chicken leg, ARP: chicken breast, PEN: whole chicken, SAU: sausage, PIP: chicken drumstick, AIP: chicken wing, QAP: chicken forequarter, API: guinea fowl wing. A, B, C, D, E, F, G: names of cold rooms.
![]()
Table 2. Escherichia coli carriage rates in samples.
Legend: CUP: chicken leg, ARP: chicken breast, PEN: whole chicken, SAU: sausage, PIP: chicken drumstick, AIP: chicken wing, QAP: chicken forequarter, API: guinea fowl wing. A, B, C, D, E, F, G: names of cold rooms.
were the most contaminated with 28/30 (93.33%). Samples from cold room A were the most contaminated with ASR at 29/35 (82.86%) (Table 3).
3.1.4. Prevalence of Staphylococci
Staphylococci were absent from all the samples analyzed (Table 4).
3.1.5. Prevalence of Salmonella
Salmonella spp. was detected in 7/285 (2.45%) of the samples: 1.82% of chicken thighs, 100% of whole chickens and 2.86% of chicken forequarters (Table 5).
3.2. Sample Contamination Levels and Declaration of Conformity
The distribution of germs by level of contamination revealed rates of 28.57% and 3.33% respectively of chicken forequarters and guinea fowl wings that were unsatisfactory with regard to sulphite-reducing anaerobes. With regard to Salmonella, 100% of Whole Chicken as well as 2.86% of Chicken Forequarters and 1.82% of Chicken Legs were Unsatisfactory with regard to compliance criteria (Table 6).
3.3. Salmonella Serotype and Antibiotic Susceptibility Profile
Serogrouping of the seven strains revealed 5 OMA strains, including one from chicken thighs, one isolated from chicken forequarters and the others from whole chickens. The other two salmonella strains were OMB and HMB, both from whole chickens. Antibiotic sensitivity tests revealed resistance to certain beta-lactam antibiotics and quinolones, in particular amoxicillin (28.57%), amoxicillin-clavulanic acid (14.28%), cefalexime (28.57%), cefoxitin (14.28%), cefuroxime (28.57%), ceftazidime (28.57%), ceftriaxone (28.57%), aztreonam (28.57%) and nalidixic acid (28.57%). However, 100% of strains were sensitive to imipenem, the latest generation of beta-lactam antibiotics (Table 7).
![]()
Table 3. Carriage rate of Anaerobic Sulphito Reducingin samples.
Legend: CUP: chicken leg, ARP: chicken breast, PEN: whole chicken, SAU: sausage, PIP: chicken drumstick, AIP: chicken wing, QAP: chicken forequarter, API: guinea fowl wing. A, B, C, D, E, F, G: names of cold rooms.
![]()
Table 4. Carriage rate of Staphylococci in the samples.
Legend: CUP: chicken leg, ARP: chicken breast, PEN: whole chicken, SAU: sausage, PIP: chicken drumstick, AIP: chicken wing, QAP: chicken forequarter, API: guinea fowl wing. A, B, C, D, E, F, G: names of cold rooms.
![]()
Table 5. Carriage rate of Salmonella spp. in the samples.
Legend: CUP: chicken leg, ARP: chicken breast, PEN: whole chicken, SAU: sausage, PIP: chicken drumstick, AIP: chicken wing, QAP: chicken forequarter, API: guinea fowl wing. A, B, C, D, E, F, G: names of cold rooms.
![]()
Table 6. Breakdown of germs isolated by level of contamination.
Legend: CUP: chicken leg, ARP: chicken armor, PEN: whole chicken, SAU: sausage, PIP: chicken drumstick, AIP: chicken wing, QAP: chicken forequarter, API: guinea fowl wing. TP: carriage rate, C: conformity, S: satisfactory, A: acceptable, NS: unsatisfactory.
![]()
Table 7. Results of antibiogram tests on isolated salmonella (n = 7).
Legend: AM: Amoxicillin, AMC: Augmentin, TC: Ticarcillin TCC: Ticarcillin + clavulanic acid, PRL: Piperacillin + Tazobactam, CL: Cefalexin, FOX: Cefoxitin, CXM: Cefuroxime, CAZ: Ceftazidime, CTR: Ceftriaxone, CEF: Cefepime, ATM: Aztreonam, IPM: Imipeneme, ETP: Ertapeneme, AK: Amikacin, GEN: Gentamicin, TOB: Tobramycin, NET: Netilmicin, C: Chloramphenicol, SXT: Trimethoprime-Sulfamethoxazole, NA: Nalidixic acid, OFX: Ofloxacin, NOR: Norfloxacin, CIP: Ciprofloxacin, FF: Fosfomycin and N: Nitrofurantoin.
4. Discussion
In Togo, despite the government’s efforts, food requirements in terms of animal proteins are not covered by national production and are subject to huge imports of meat products. It is therefore imperative to examine the microbiological quality of broiler meat in order to assess the risk it may pose to public health. The table “Table 8” and “Table 9” below summarize the prevalence, average loads and base 10 log expressions obtained in this study.
In this study, qualitative and quantitative analysis revealed that 71.71% of the samples were contaminated with TAMF, with an average load of (5.92 log10 CFU/g). This could be mainly due to the lack of hygiene of the processes in the slaughterhouses of origin. Our results are similar to those obtained by Omorodion et al. (2014) [12] and L. Kozačinski et al. (2006) [13] with respective loads of 5.96 log CFU/g and 5.23 log10 CFU/g in market chicken meat. In contrast to our study, Bhandari et al. (2013) [14] , Sengupta et al. (2012) [15] , found respective loads of 7.24 log, CFU/g, 6.39 log CFU/g as well as 'Abraham Adu-Gyamfi et al. (2012) [16] who in a study of chickens in supermarkets, local markets and farms, obtained respective averages of 6.46, 6.91 and 6.57 log10 CFU/g. In Korea, the average levels of TAMF found in chicken samples were 3.10 log CFU/g [17] , which is lower than in the present study (5.92 log CFU/g). Also, low loads of TAMF compared to our results were reported by Siddiqui et al. (2008) (3.67 log CFU/g) [18] ; Al jasser et al. (2012) (4.03 log CFU/g) [19] and Fernandes et al. 2016 (4.15 log CFU/g) in Brazil [20] .
Despite the 71.71% prevalence of TAMF and a mean load of 5.92 log CFU/g, all the samples submitted to our study were downgraded to Satisfactory according to the FCD 2022 [10] criteria and the three-class design analysis. In contrast,
![]()
Table 8. Prevalence, average load and expression in log 10 of the results obtained.
Table 9. Summary of the average loads of all the germs tested for according to the different types of samples.
Parameters
Criteria
CUP (55)
ARP (10)
PEN (5)
SAU (30)
PIP (95)
AIP (25)
QAP (35)
API (30)
ASR
≤m
≤100
<10
<10
<10
<10
<10
<10
(24) <10
(15) ≤100
m < x < M
100 < x < 103
(1) 2.6 × 102
(14) 3.3 ± 2.6 × 102
M
M˃103
(10) 1.1 ± 1.05 × 103
(1) 1.3 × 103
AMTF
≤m
<5 × 106
<5 × 106
8.3 × 106 ± 4.7 × 106
<5 × 106
(3) <1.0 × 105
3.6 × 105 ± 3.6 × 104
(27) <1 × 105
5.8 × 105 ± 3.5 × 105
m < x < M
107 < x < 108
(92) 4.5 ± 2.9 × 106
(8) 5.3 × 105 ± 4.9 × 105
M
Escherichia coli
≤m
(43) <100
<100
<100
<100
<400
<400
(16) <100
(1) <400
m < x < M
(12) 1.1 × 104 ± 7.6 × 103
(19) 1.1 × 104 ± 7.6 × 103
(29) 1.9 × 103 ± 1.3 × 103
M
Staphylococci
≤m
<100
<100
<100
<100
<100
<100
<100
<100
<100
Salmonella
ND
(54) ND
ND
(5) D
ND
ND
ND
(34) ND
ND
D
(1) D
(1) D
Legend: CUP: chicken leg, ARP: chicken breast, PEN: whole chicken, SAU: sausage, PIP: chicken drumstick, AIP: chicken wing, QAP: chicken forequarter, API: guinea fowl wing. A, B, C, D, E, F, G: names of cold rooms. D: Detected, ND: Not Detected; m = the criterion and M = 10 m.
a similar study carried out in Casablanca, Morocco, revealed that 29.2% of all poultry meat samples tested had an unacceptable TAMF according to Moroccan regulations [21] . Our results are also contrary to those of GÜRAN et al. (2019) in Turkey, who on 240 samples of organic frozen chicken meat were 100% unsatisfactory with an average AMTF in chicken pieces (breast, thigh, chicken wings) of 4.99 ± 0.80 log10 CFU/g [21] [22] .
It should be mentioned that TAMF can predict the shelf life of food products and is mainly used as an indicator of hygiene and process quality but not safety. TAMF in raw poultry indicates the hygienic conditions of the processing plants in which the food is treated and a high load increases the risk of microbial spoilage of the products [21] [22] .
Staphylococci were absent from all the samples analyzed in our study. In contrast to our results, Maharjan et al. (2019) [23] note the presence of 17.1% Staphylococci aureus in market meat; Kozačinski et al. (2012) (17.9%) [13] and Khallaf et al. (2014) (16.66%) [24] . This difference could be explained by the absence of this germ throughout the production chain. Indeed, Khallaf et al worked on chickens taken from slaughterhouse sites with different levels of hygiene. Thus, the degree of hygiene in slaughterhouses, the cleanliness of the water used during scalding, the hygiene of staff and the precautions taken during evisceration may be risk factors for contamination of chickens. Also, relatively high prevalence has been obtained by, Lidija Kozačinski, et al., (2006) [13] ; Yar et al. (2021) [25] and N. Alloui et al. (2013) [8] respectively 30.30%; 35.4% and 46.66%. In other studies, Jansen et al (2018) (95%) [26] and Saikia et al. (2010) (100%) [27] obtained higher prevalence of Staphylococcus aureus in meat. These total Staphylococci were found with a load of 1.99 log CFU/g by Maharjan et al. (2019) [23] in market meat and also in studies carried out by Sengupta et al. (2012) (3.7 log CFU/g) [15] and Saikia et al. (2010) (4.07 log CFU/g) [27] .
The presence of staphylococci in meat reflects unsanitary conditions, cross-contamination between the processing phase and the surrounding environment, processing temperature and personal contact. Staphylococcus aureus is a commensal organism of human skin and also a common pathogen, causing minor to severe infections, including food poisoning [28] .
Escherichia coli, which is an indicator of fecal contamination, synonymous with a lack of hygiene in the production process, gave a prevalence of 32.98% with an average load of 3.85 log10UFC/g during our study. A similar prevalence was obtained by Yar et al. (2021), 26.2% [25] . Our results are lower than those obtained by N. Cohen et al. 2007 (48.4%) [21] ; Jansen et al. 2018 (67%) [26] and Mahmoud et al. (2020) [29] , (54% and 48%) in chicken thighs. Compared to the average load, the mean numbers of Escherichia parcel detected in supermarkets, local markets and farms in a study carried out in Accra, Ghana by Abraham Adu-Gyamfi et al. (2012) [16] are lower than ours (3.85 log10 CFU/g) and were 1.27, 2.59 and 2.74 log10 CFU/g respectively.
In contrast to our study, high prevalence was obtained by Egervärn et al. (2014) (95%) in poultry meat imported mainly from Brazil (n = 40) in Sweden [30] . This difference in prevalence could be explained by the number of samples tested in these studies and ours and also by the fact that Escherichia coli is a mesophilic germ whose growth is inhibited by negative cold. Chicken meat is one of the main sources of good quality protein, but it is also sensitive to microbial contamination and is often implicated in food-borne illness. The presence of Escherichia coli is an important indicator of pollution and is the food-borne pathogen of greatest concern for public health [31] .
ASR were found in our study with a prevalence of 14.73% and a mean load of 2.98 lo10UFC/g. Our results are superior to those obtained by L. Kozačinski al.:2006 [13] and Khan et al. 2015 [32] ; who find prevalence of (1.50%) and (6%) in chickens, respectively. A contrary trend was recorded by Miwa et al. with a relatively high prevalence (82% - 84%) of Clostridium perfringens in poultry meat compared to beef (16%) and pork (10%) (Miwa et al. 1998) [33] . In contrast to our study, a high prevalence was obtained by Singh et al. (2005) [34] . According to Billon, these microorganisms are telluric germs, present in the intestines of animals and also found in humans, and can be responsible for toxic infections when the number of spores (genus clostridium) contained in the food exceeds the criteria [35] . Salmonella spp. was detected in 7/285 (2.45%) of the samples in our study. Similar results were obtained by: N. Cohen et al. (2007) (1.6%) [21] in Morocco and Jansen et al. 2018 (1.2%) [26] . Our results are inferior to those obtained by L. Kozačinski et al. (2006) [13] , Adeyanju and Ishola (2014) [36] , Fernandes et al. (2016) [20] , Mahmoud et al. (2020) [29] ; respectively 10.60%; 33%; 18% and 9% in similar studies on frozen chicken. Contrary to our study, high prevalence was obtained by N. Alloui et al. (2013) [8] 83.33% and 73.33% by Elgroud et al. in 2009 [37] . Also, the prevalence of Salmonella in chicken and meat in slaughterhouses in Thailand and Japan was 41.2% and 40.7%, respectively [38] .
This prevalence, whatever their values, are alarming because Escherichia coli and Salmonella are the most important and most frequent pathogens responsible for food poisoning and food-related infections in chicken meat [39] [40] . Similarly, breaded products based on frozen reformulated chicken have already been implicated in cases of salmonellosis in humans. An epidemic of Salmonella enterica serovar Enteritidis involving several strains and more than 400 human cases was reported in the UK in 2020 [41] . It should be noted that poultry can act as a reservoir for Salmonella and cause food-borne infections in humans [42] .
However, strict animal hygiene can reduce the number of contaminated carcasses. Therefore, the lack of strict hygiene throughout the production chain, the absence of health monitoring programs and the non-compliant prophylactic use of antibiotics against Salmonella could explain the presence of these Salmonella in these samples.
In this study, in terms of hygienic quality, all the samples were downgraded to satisfactory in terms of AMTF and Staphylococcus [10] . 28.57% and 3.33% of chicken forequarters and guinea fowl wings respectively were Unsatisfactory with respect to reducing sulphur anaerobes. With regard to Salmonella spp, 1.82%, 2.86% and 100% of chicken thighs, chicken forequarters and whole chicken, respectively, were contaminated (Table 6 and Table 9). Certain rules of good hygiene and manufacturing practice, such as conditions of slaughter, plucking, evisceration, bleeding, washing, rinsing, preservation and sale, are often not respected during the slaughter process. Non-compliance with these hygiene rules is generally thought to be the cause of meat contamination.
In relation to Salmonella antibiograms, 28.7% (2/7) of Salmonella spp. (AOM) isolated were resistant to the following molecules: amoxicillin, amoxicillin-Ac clavulanique, cefalexime, cefuroxime, ceftazidime; aztreonam, nalidixic acid, ceftriaxone and 14.28% (1/7) were resistant to cefoxitin. All the other molecules tested showed no resistance. These tests revealed the presence of an ESBL strain (Amoxicillin-R, Amox + clav.-R, Cefalexin-R, Cefoxitin-S, Ceftriaxone-R, Cefepime -S), so we observed a “champagne cork” synergy between the amoxicillin + Clavulanic acid disc and the Ceftriaxone disc or another C3G/C4G. We also observed a cephalosporinase hyperproducing strain (Amoxicillin-R, Amox+clav.-R, Cefalexin-R, Cefepime -S). R, Cefalexin-R, Cefoxitin-R, Ceftriaxone-R, Cefepime -S).
According to the 2023 veterinary recommendations [11] of the CA SFM: 1) If an extended-spectrum beta-lactamase (ESBL) is detected, the strain must be considered resistant to all beta-lactams available in veterinary medicine, with the exception of the amoxicillin-clavulanic acid combination. For this antibiotic, the raw result (S, I or R) is not subject to this interpretation rule. However, the in vivo efficacy of amoxicillin/clavulanic acid on a strain with an ESBL has not been documented in veterinary medicine. 2) In the event of evidence of cephalosporinase hyperproduction, the strain should be considered resistant to all beta-lactam antibiotics available in veterinary medicine [11] . However, the two ESBL and cephalosporinase hyperproducing strains in our study are resistant to all beta-lactams. These results confirm that, in poultry, these drugs are used either to treat disease or as non-prescription growth promoters, as they are cheap and readily available. Similar results showing resistance to Cefotaxime have been obtained from local chicken products by Sasaki et al. (2021) [43] and by other studies in Japan and Brazil [44] [45] . Our results (28.7%) differ from those obtained by Caroline Bouda in Burkina Faso, who found resistance of around 60% to amoxicillin-clavulanic acid in chicken and guinea fowl isolates [46] . Similar studies have shown that the increase in antimicrobial resistance among Salmonella strains in recent years can be attributed to the selection pressure created by the inappropriate application of antibiotics in veterinary and human medicine [47] .
We can extrapolate from the resistance observed to nalidixic acid by concluding that these two strains are then resistant to Fluoroquinolones, as nalidixic acid is the best marker of the first levels of resistance to quinolones [11] . In parallel with our study, very high levels of resistance to nalidixic acid (72.3%) were observed in a study in China [48] and in Brazil; in a comparative study between 2014 and 2017, with respective rates of 57.5% and 86.5% [49] .
Sasaki et al. (2021) observed different high rates of resistance from us for streptomycin (51.1%), tetracycline (33.1%) and kanamycin (18.4%) [43] . However, the high sensitivities that we observed to the molecules tested could be explained either by the low use or the non-use of these antibiotics in poultry farms today. This difference in results with these authors can also be explained by the current awareness among farmers of the overuse of antibiotics, as these antibiotics are used haphazardly on farms and inevitably create selection pressure that favors the development of multi-resistant bacteria.
This multi-resistance is much more worrying, as these are antibiotic molecules commonly used in the treatment of human salmonellosis. In recent years, antimicrobial resistance in Salmonella spp. has increased due to factors such as the widespread use of antimicrobial agents in the food industry and veterinary medicine, growth-promoting substances and prophylactic and therapeutic solutions [50] [51] .
However, this undesirable trend has caused concern in the scientific community, as it not only increases the difficulty of treating salmonellosis, but also the spread of antimicrobial resistance in the food chain, particularly in chickens and poultry products [50] [52] [53] .
5. Conclusions
Non-compliance with hygiene rules and poor preservation are generally thought to be the cause of contamination of chicken meat. The use of antimicrobials in food-producing animals can lead to increased bacterial resistance. It has been shown that some of the multi-resistant Salmonella strains found in humans are of animal origin and have acquired their resistance genes on farms before being transmitted to humans via food. Monitoring antimicrobial resistance in food animals can provide important information to address this problem.
The present study was undertaken to fill this gap and contribute to the development of antimicrobial resistance control strategies in Togo. It has enabled the acquisition of data on the hygienic quality of imported chickens and bacterial resistance to antibiotics, which is necessary for better therapeutic management of infections and also contributes to the development of a strategy for controlling antimicrobial resistance.
Ethical Considerations
Permission to conduct this study was obtained from the poultry carcasses sellers and the study protocol was approved by the Ethical Committee of Togo.