Characterization and Structural Studies of Metal Complexes of 5-Aminomethyl-[13]aneN4 ()

1. Introduction
Numerous studies have been conducted on cyclic tetraamines and their coordination properties with various metal cations, which enable their use in numerous fields, such as ultrapurification of liquids or selective fixation of gases [1] [2] and they are known for their complexation properties regarding transition and heavy metals [3] [4] . The medical sector also utilizes metal complexes of these nitrogenous cyclic ligands, as contrast agents in magnetic resonance imaging or in nuclear medicine [5] [6] [7] , development of agents for biotechnology [8] , nuclear Medical Applications [9] and for biomedical application [10] . This multiple application is explained by the complexing properties of polyazacycloalkanes which are controlled by the size of the macrocycle.
The C-functionalized cyclic polyamines possess the ability to introduce one or more functionalities into the molecule while keeping the four secondary amine functions of the macrocycle intact. Indeed, these macrocycles make it modulable for the selectivity towards metal cations. The majority of studies have focused on C-functionalized cyclam compound ligands for metal complexation because they have an affinity for complex metals as a result of their tendency to form stable and inert chelates when necessary [4] [11] [12] [13] [14] . Furthermore, the inclusion of pendant arms with additional donor groups frequently results in complexes with high thermodynamic stability and even inertness when dissociated.
Metals are elements present in the environment as well as in living species. Some metals are essential in the processes occurring in living organisms and in the enzymatic reactions of the human body such as nickel, copper and zinc, other metals are harmful to the environment and to human health, such as heavy metals such as chromium. Our focus was on studying how the macrocycle 5-aminomethyl-[13] aneN4 complexes metals like copper, chromium, and nickel.
In this work, we have resynthesized an N-functionalized macrocycle which has already been developed by our team [15] , in order to be able to complex it with the following metals: Ni2+, Cu2+ and Cr3+. To determine how metals are coordinated by macrocycle, particularly if the primary amine coordinates the metal ion, this complex was characterized by X-ray diffraction, UV-Visible spectrometry and electron paramagnetic resonance (EPR) for the copper complex.
2. Materials and Methods
2.1. Synthesis of cis-(9b,9c-Dimethyldecahydro-2a,4a,7a,9a-tetraazacyclopenta[cd]-phenylen-1-yl)methanamine (Ligand 1)
A solution of 2,3-butanedione (27.45 g, 0.319 mol) in acetonitrile (10 mL) was added to a solution of N,N-bis(aminoethyl)propane-1,3-diamine (51.1 g, 0.319 mmol) in acetonitrile (1.5 L) at 0˚C. The mixture was stirred at this temperature for 2 h. Benzotriazole (38.1 g, 1 equiv.) and K2CO3 (88.2 g, 2 equiv.) were added. A solution of 50% chloroacetaldehyde in water (50.1 g, 0.319 mol) was slowly added at 0˚C and the resulting mixture was stirred overnight at room temperature. Then the solution was filtered throught Celite and washed with acetonitrile (100 mL). The filtrate was evaporated. The resulting solide was dissolved in CH2Cl2 (500 mL). After filtration, the organic phase was washed with a 3 M NaOH solution (200 mL). After extraction, the organic phase was dried with MgSO4 and the solvent was evaporated. The residual brown solid was purified by aluminium oxide chromatography (eluent: CH2Cl2). A solution of this compound (40.73 g, 0.156 mol) in dry THF (50 mL) was slowly added to a suspension of LiAlH4 (11.8 g, 0.31 mol) in THF (200 mL) under nitrogen at −78˚C. The resulting mixture was stirred overnight. Ethyl acetate (100 mL) and then water (25 mL) were carefully added. After removal of the solvent, the residual white-grey solid was taken up in chloroform (2 × 200 mL) and insoluble products were eliminated by filtration. Compound 1 was obtained as a colorless oil (yield 32.16 g, 78%). 1H NMR (300 MHz,CDCl3, 300 K): δ = 1.04 (s, 3 H), 1.12 (s, 3 H), 1.27 (s, 2.9 H), 1.28 (s, 2.9 H), 1.76 (m, 1.3 H), 2.2 - 3.6 (m, 26.6 H) ppm. 13C{1H} NMR (75 MHz, CDCl3, 300K): δ = 11.9, 12.3 (×2), 13.4 (CH3), 18.7, 25.8 (CH2-β), 44.8, 44.9, 45.7, 45.9, 46.5, 46.6, 47.2 (×2), 48.0, 48.3, 48.4, 49.4, 50.0, 50.1, 51.0, 51.1 (CH2-α), 61.8, 68.1 (C-H), 73.2, 73.4, 79.3, 80.5 (N-C-N) ppm. MS (MALDI-TOF): m/z = 265.82 [M]+.
2.2. Synthesis of (1,4,7,10-Tetraazacyclotridecan-5-yl)methanamine (Ligand 2)
A solution of 35% hydrochloric acid (107 mL, 1.2 mol) was added to a solution of 1 (32.16 g, 0.12 mol) in ethanol (200 mL). The resulting mixture was heated at reflux for 4 h. After cooling, the solution was filtered and washed with ethanol (50 mL) and then diethyl ether (100 mL). The solid was dissolved in a saturated 15 M NaOH solution (10 mL). After extraction with chloroform (2 × 150 mL), the organic phase was dried with MgSO4 and the solvent was evaporated. Compound 2 was obtained as a white solid; yield 11.02 g, 42%. 1H NMR (300 MHz, CDCl3): δ = 1.63 (m, 2 H), 1.87 (s, 6 H), 2.67 - 2.76 (m, 17 H) ppm. 13C{1H} NMR (75 MHz, CDCl3): δ = 28.9 (CH2-β), 44.3, 46.2, 47.7, 49.0, 49.0, 49.7, 49.8, 50.8 (CH2-α), 59.0 (CH)ppm. MS (MALDI-TOF): m/z = 215.68 [M]+. C10H25N5∙0.2H2O (221.62): calculated. C 54.86, H 11.69, N 31.99; found C 55, H 11.57, N 31.81.
2.3. Synthesis of [Ni(2)](ClO4)2
Ni(ClO4)2. 6H2O (385 mg, 1.05 mmol) is added to a solution of compound 2 (215 mg, 1.05 mmol) in water (10 mL). The mixture is stirred at 60˚C for 2 h. The solution is concentrated then the pH is adjusted to 9 by adding NaOH (0.1 M). The diffusion of propanol into the preceding solution makes it possible to recrystallize the product in the form of purple crystals (359mg, 0.62 mmol, Yield = 62%). MALDI-TOF: m/z = 372.75 [M-ClO4]+; 372.09 calculated for C10H25ClN5NiO4. UV-vis. (CH3OH): λmax/nm (ε/M−1 cm−1) = 516 (73), 385 (160). Elemental analysis C10H25N5Ni(ClO4)2. 2H2O: calculated: C 23.6; N 13.76; H 5.74; found: C 23.98; N 13.80; H 5.23.
2.4. Synthesis of [Ni(2)](C2H3O2)2
Ni(C2H3O2)2∙4H2O (577.35 mg, 2.32 mmol) is added to a solution of compound 2 (500 mg, 2.32 mmol) in methanol (20 mL). The mixture is brought to reflux for 2 h then evaporated. The purple solid is redissolved in acetonitrile and then filtered. The violet-colored complex is obtained by evaporation of the solution (725 mg, 1.85 mmol, Yield = 80%). MALDI-TOF: m/z = 273.83 [M-2C2H3O2]+; 273.15 calculated for C10H2N5Ni. UV-vis. (CH3OH) λmax/nm (ε/M−1cm−1) = 543 (14), 340 (42). Elemental analysis C10H25 N5Ni(C2H3O2)2. 2H2O: calculated: C 39.27; N 16.36; H 8.24; found: C 39.87; N 16.36; H 8.24.
2.5. Synthesis of [Cu(2)]Cl2
CuCl2 (140 mg, 0.93 mmol) is added to a solution of compound 2 (200 mg, 0.93 mmol) in methanol (30 mL). The mixture is brought to reflux for 3 h. The final blue colored compound is obtained by precipitation in diethyl ether (299 mg, 0.855 mmol, Yield = 92%). MALDI-TOF: m/z = 278.75 [M-2Cl]+; 278.14 calculated for C10H25N5Cu. UV-vis. (CH3OH): λmax/nm (ε/M−1cm−1) = 582 (145). Elemental analysis C10H25N5CuCl2∙3H2O: calculated: C 29.74; N 17.30; H 7.74; found: C 29.97; N 17.20; H 7.30.
2.6. Synthesis of [Cr(2)]Cl3
CrCl3(THF)3 (1.13 g, 3 mmol) is added to a solution of compound 2 (0.76 g, 3.53 mmol) in DMF (7 mL). The mixture is brought to reflux under nitrogen for 50 min. After adding acetone (7 mL), the precipitate is filtered off and then washed with acetone (2 × 7 mL) and diethyl ether (2 × 7 mL). The expected complex is obtained in the form of purple crystals after recrystallization from methanol (1.06 g, 2.85 mmol, Yield = 81%). MALDI-TOF: m/z = 302.65 [M-2Cl]+; 302.12 found for C10H25ClCrN5. UV-vis. (CH3OH): λmax/nm (ε/M−1cm−1) = 537 (331), 384 (280), 330 (810). Elemental analysis C10H25Cl3CrN5∙H2O: calculated: C 30.94; N 17.12; H 6.87; found: C 30.66; N 17.88; H 6.95.
3. Results and Discussion
3.1. Synthesis of the Ligand (2)
The C-functionalization of [13]aneN4 by an amine group was carried out by Yoann Rousselin et al. [15] The condensation of the butanedione with the tetraamine 232 enables the bisaminal derivative to be obtained. The cyclization was carried out using chloroacetaldehyde as a biselectrophilic synthon in the presence of potassium carbonate and benzotriazole. A nucleophilic substitution of the benzotriazole group by the cyano group allows compound 1 to be obtained with a yield of 70%.
Next, the reduction of the nitrile group to a primary amine is carried out by adding lithium tetrahydruroaluminate. The bisaminal bridge is removed in an acidic medium to give access to compound 2 with an overall yield of 42% (Scheme 1).
Compound 2 was recrystallized and the crystallographic structure was obtained by X-ray diffraction (Figure 1).
In the structure of this compound, we observe the presence of an intramolecular hydrogen bond N1-H4b. NC bond lengths vary between 1.457 and 1.509 Å (N1-C1: 1.472 Å; N1-C9: 1.457 Å; N2-C2: 1.509 Å; N2-C3: 1.508 Å; N3-C5: 1.494 Å; N3-C6: 1.487 Å; N4-C7: 1.476 Å; N4-C8: 1.463 Å and N5-C10: 1.491 Å). The values of Macrocycle N-C bond lengths are similar to those obtained for [13]
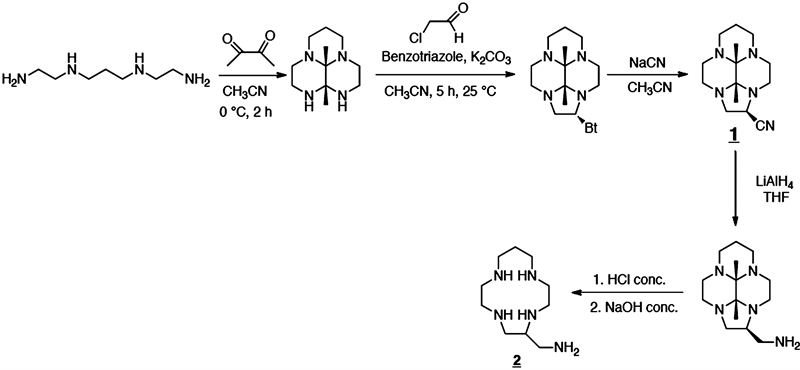
Scheme 1. Synthesis of (1,4,7,10-Tetraazacyclotridecan-5-yl)methanamine (Ligand 2).
![]()
Figure 1. ORTEP view of compound 2. The ellipsoids are represented at the 50% probability level.
aneN4 (1.49 to 1.51 Å) [16] . Table 1 shows the crystallographic data for the ligand 2.
3.2. Physico-Chemical Characterization of Metal Complexes
3.2.1. Characterization of Ni2+ Complexes
The crystallographic structure of the complex of Ni(II) by compound 2 was
![]()
Table 1. Crystallographic data for the ligand 2.
obtained after recrystallization by diffusion of propan-1-ol in water. An ORTEP view of this structure is shown in Figure 2.
In the [Ni(2)] (ClO4)2 complex, the metal ion Ni2+ is hexacoordinated by five nitrogen atoms of the macrocycle and one oxygen atom of a perchlorate ion, with an octahedral geometry. One of the nitrogen atoms of the macrocycle and the oxygen atom are in axial positions. The other three nitrogen atoms of the macrocycle as well as the nitrogen atom of the primary amine function are in the equatorial plane. The bond lengths between nickel(II) and nitrogen atoms vary between 2.03 and 2.11 Å (Ni-N1 = 2.11 Å, Ni-N3 = 2.09 Å, Ni-N5 = 2.10 Å and Ni-N2 = 2.04 Å) and the length of the axial bond Ni-N4 = 2.07 Å. For greater clarity, we have represented the octahedron formed around the metal (Figure 3). Table 2 shows the crystallographic data for [Ni(2)](ClO4)2 complex.
![]()
Figure 2. ORTEP view of the [Ni(2)](ClO4)2 complex. Ellipsoids are shown at the 50% probability level. Hydrogen atoms have been omitted for clarity.
![]()
Figure 3. View of the coordination polyhedron in the [Ni(2)](ClO4)2 complex.
![]()
Table 2. Crystallographic data for [Ni(2)](ClO4)2 complex.
The spectra in solution of this complex are recorded between 334 and 614 nm in methanol. In Figure 4 we represent the UV-visible spectrum of the complex [Ni(2)](C2H3O2)2.
The maximum of the absorption bands corresponding to the d-d transitions in the complex [Ni(2)](C2H3O2)2 is located at wavelengths of 340 and 543 nm (ε = 42 and 14 M−1∙cm−1), which is characteristic of a hexacoordinated nickel(II) complex in an octahedral geometry (Ni2+ high-spin) [16] .
A UV-visible study of this complex was performed in water (KCl, 0.1 M) as a function of pH. The band due to the d-d transition centered at a wavelength 425 nm in an acidic environment (2 < pH < 5) is characteristic of a low-spin nickel of plan-square geometry. Above pH 5, the absorption band is observed at a wavelength 538 nm (which does not change even at pH 12) characteristic of the octahedral coordination geometry of a nickel complex (II) [17] .
This change in coordination geometry is observed by colorimetry. Indeed, we were able to distinguish the change in coloration of the Ni2+ complexes as a function of the pH: from the yellow color at acidic pH to purple color at basic pH (Figure 5).
3.2.2. Characterization of Cu2+ Complexes
Our team characterized the crystallographic structure of [Cu(2)]2+ and it is shown in Figure 6 [18] .
In this complex, the copper is pentacoordinated and presents a pyramidal geometry with a square base. The four nitrogen atoms of the macrocycle and the metal center are in the equatorial plane. The primary amine of a neighboring macrocycle coordinates at the apical position, allowing the formation of a coordinating polymer. Bond lengths between the copper(II) atom and nitrogen atoms vary between 2.025 and 2.199 Å [18] .
![]()
Figure 4. UV-visible spectrum of the [Ni(2)](C2H3O2)2 complex recorded in methanol.
![]()
Figure 5. Change in color of aqueous solutions of Ni2+ complexes as a function of pH.
The UV-visible spectra of the copper(II) complex are recorded in a solution of methanol. The maximum absorption of the broadband corresponding to the d-d transitions is observed in λ = 582 nm (ε = 145 M−1∙cm−1), this value is consistent with a pentacoordinated metal center [19] [20] .
We carried out UV-visible studies as a function of the pH of this complex in H2O (KNO3, 0.1 M) using HNO3 (0.1 M) as the acid and NaOH (0.1 M) as the base. The superposition of the absorption spectra does not show a displacement of the copper d-d transition band but an increase in the molar extinction coefficient as the pH of the medium increases. This increase in the molar extinction coefficient can be explained by a low disturbance of the structure of the complex (while keeping the same coordination geometry). In this case, two hypotheses can be put forward:
In a basic environment, the amine function coordinates on the metal center. On the other hand, in an acidic environment, the amine protonates, causing it to move away from the copper and consequently, a molecule of the solvent coordinates on the metal.
The nitrogen atom of the amine group does not coordinate with copper at basic pH and the hyperchromic effect is due to the deprotonation of the water molecule coordinated with the metal center in the axial position.
The RPE spectra of this complex are recorded in X-band, which is to say at a frequency of about 9.3 GHz in frozen solution at 100 K in a methanol/toluene mixture (2/1). The values of the parallel and perpendicular components (g//, g⊥) and of the hyperfine coupling constant (A//) are reported in Table 3.
![]()
Figure 6. ORTEP view of the copper(II) complex. Ellipsoids are shown at the 50% probability level. Hydrogen atoms have been omitted for clarity [18] .
![]()
Table 3. Values of g⊥, g// and A// of the complex of [Cu(2)]2+ measured on the spectra RPE.
The spectrum is characterized by a wide line associated with values of g⊥ in 2068 and four equidistant lines at weaker fields associated with value of g// in 2.18. The bursting of the g// component into four lines results from the hyperfine interaction with the copper nucleus of nuclear spin I = 3/2.
Likewise, the position of g// with respect to g⊥ on the spectrum reflects the nature of the orbital which is occupied by the free electron. When the value of g// is greater than g⊥, the single electron is located in the dx2−y2 orbital [20] . The values of g// and g⊥ correspond to complex of Cu2+ having a geometry pyramidal with a little distorted square base or an octahedral geometry axially elongated by the “Jahn-Teller” effect.
Spectra relating to mid-field transitions around 1600 Gauss were recorded for this complex at high enough concentrations in order to characterize the presence of forbidden spin transitions (ΔMs = 2), the value obtained (g// = 4187) can show a dipolar coupling between two adjacent Cu2+ ions.
3.2.3. Characterization of Cr3+ Complexes:
The crystals complex of the [Cr(2)]Cl3 was obtained by recrystallization in methanol (Figure 7).
In this complex, chromium is hexacoordinated in an octahedral environment. One of the macrocycle’s nitrogen atoms and one chlorine atom are in apical positions. The other three nitrogen atoms of the macrocycle as well as the nitrogen atom of the primary amine function are in the equatorial plane. The lengths of the Cr-N bonds vary between 2.06 and 2.09 Å. In complexes of chromium with tetraazacycloalkanes described in the literature, the metal is always hexacoordinated,
![]()
Figure 7. ORTEP view of the chrome complex. Ellipsoids are shown at the 50% probability level. Hydrogen atoms have been omitted for clarity.
[21] [22] but the plane of the octahedron is generally formed by the four nitrogen atoms of the ring and the chlorine atoms are in position apical. For greater clarity, we have represented the octahedron formed around the metal (Figure 8). Table 4 shows the crystallographic data for [Cr(2)]Cl3 complex.
![]()
Figure 8. View of the coordination polyhedron in the complex.
![]()
Table 4. Crystallographic data for [Cr(2)]Cl3 complex.
![]()
Figure 9. ORTEP view of the complex of: (a) nickel, (b) chromium.
The comparison of the crystallographic structures of the [Cr(2)]Cl3 and [Ni(2)](ClO4)2 complexes shows that the two metal cations have the same coordination geometry. N-M bond lengths (M: metal) in Å are shown in Figure 9.
The ionic radius of the Ni2+ ion (0.069 nm) is greater compared to the Cr3+ ion (0.061 nm). We expect the N-Cr bond lengths to be longer than those of N-Ni. However, this is only verified for the case of the N2-M bond.
The maximum of the absorption bands corresponding to the d-d transitions in this complex is located at wavelengths of 537 nm (ε = 331 M−1∙cm−1) which is characteristic of a hexacoordinated chromium(III) complex [23] [24] . The molar extinction coefficients of chromium complexes generally distinguish the cis and trans isomers of chromium(III) [21] . The cis complexes of this metal cation often have higher extinction coefficients than those corresponding to the trans isomer, which can explain that the complex has a cis conformation.
4. Conclusion
In this work, we tried to study the coordination of some metals like Cu(II), Ni(II) and Cr(III) by a C-functionalized macrocycle 5-aminomethyl [13]aneN4. The characterization of the metal complexes of this ligand made it possible to show that the primary amine function completes the coordination sphere of the metal center. Indeed, the crystallographic structures of the chromium(III) and nickel(II) complexes show that the metal center is hexacoordinated in an octahedral geometry, one of the nitrogen atoms of the macrocycle and a counterion are located in the apical position. The other three nitrogen atoms of the macrocycle as well as the nitrogen atom of the primary amine function are in the equatorial plane. The M-N bond lengths vary from 2.06 to 2.09 Å for chromium and from 2.04 to 2.10 Å for nickel. In contrast, in the copper(II) complex, the metal is pentacoordinated in a square-based pyramidal environment. The four nitrogen atoms of the ligand form the base of the pyramid, and the amino arm of a neighboring molecule coordinates the metal in the apical position. This assembly leads to the formation of a one-dimensional organometallic network.
Acknowledgments
This work was supported by the CNRS, the French Minister for Research, and the Regional Council of Burgundy.