Thermal Characteristics of Earth Blocks Stabilized by Rice Husks ()
1. Introduction
Today, the construction of buildings with conventional materials such as steel and cement has become costly for developing countries like Chad, but also a source of environmental pollution because of the carbon dioxide (CO2) they emit during their production and transport. Buildings constructed with cement require enormous amounts of energy for their thermal comfort [1] [2] . Climate change leads to temperature variations from which the inhabitants must be protected by constructing buildings with low thermal conductivity materials.
Several works have already been carried out on: improving the thermal comfort of earthen buildings, determining the thermal characteristics of earthen blocks stabilised with cement and natural fibres. Bal et al. [3] worked on the dependence of water content on the thermal capacity of laterite bricks with the addition of millet waste and reported the authors’ work [4] [5] [6] [7] [8] . In their work, they studied: the impact of wood content on the thermal properties of cement-wood-clay composites; the thermophysical properties of building materials, including stabilized soils. They also determined: the thermophysical characteristics of low-cost building materials; the development of a fibre-based soil-cement block with low thermal conductivity and the thermal conductivity of cement-stabilised laterite bricks with added shells. Bobet O [9] conducted studies on the characterization of bio-sourced materials based on clay and peanut shell. Ouédraogo et al. [10] have worked on the physical, thermal and mechanical properties of adobes stabilized with fonio straw (Digitariaexilis). The latter two authors focus their thermal studies solely on the thermal conductivity of composites, but their results are not transposable, given that the results depend on soil class and plant fiber type. However, to our knowledge, very little work has been done on the thermal characterization of clays reinforced with rice husks. The measurements of the thermal properties of fired bricks have been carried out based on the various techniques of thermal metrology developed by Laaroussi et al. [11] [12] and by Lamrani et al. [13] . The objective in this work is to carry out an experimental study to measure both the thermal properties of composites based on two clay soils and rice husks with different contents, whose mechanical properties have been presented by Mbairangone et al. [14] . The special feature of our study is that the thermal parameters are measured simultaneously, rather than being calculated using mathematical formulae. Moreover, to our knowledge, this rice husk-clay mixture has not been studied in the same proportions.
In this study we will try to highlight [15] will be used to measure the conductivity, effusivity, temperature, and thermal conductivity of the composite.
2. Materials and Methods
2.1. Materials Used
The materials used in this study are clay soil and rice husks. The clay soil designated by NDJA is taken from one of the brick factories of Ndjamena, the capital city of Chad (12˚47.001''N 15˚2'57.001''E) and the one designated by MOD is taken from one of the brick factories of Moundou in the southwest of Chad (8˚34'0.001''N 15˚4'59.999''E). The rice bales come from the rice fields of Mara, a village located in the 1st district of the city of N’Djamena. The geotechnical study of these soils was carried out at the Civil Engineering Laboratory of National High School of Public Works of N’Djamena. We found that, the MOD is class A2 low plastic clay and the NDJA is low plastic clay (A2/A3). The respective organic matter contents are 5.11 and 2.71. The visual appearance of these materials is shown in Figure 1.
2.2. Preparing and Manufacturing of Adobe Samples
2.2.1. Preparation of the Clay-Rice Husk Mixtures
The clay samples were sun-dried, crushed and passed through a standard 5 mm sieve. The rice husks are those that pass through a standard 1.25 m sieve. These two materials were first dry-mixed in varying proportions according to Table 1 and Table 2, and then kneaded for 10 - 12 mm with water to obtain a malleable composite. The different compositions of the clay-rice husk mixtures produced in this way make it possible to follow and compare the evolution of their thermal properties. The proportions of these two materials are those already the evolution of the thermophysical parameters of these composites, as a function of the rice husk content and as a function of their maturation time. The asymmetric hot plane method developed by Jannot et al. [15] and further developed by Jannot [16] .
![]()
Figure 1. Rice husks and soils of Moundou (MOD) and N’Djamena (NDJA).
![]()
Table 1. Composition of soil mixtures MOD.
![]()
Table 2. Composition of soil mixtures NDJA.
Used in the adobes whose mechanical characteristics are the subject of the article [14] . It can be seen from Table 1 and Table 2 that the water content of the MOD soil is slightly higher than that of the NDJA soil, as the structure of the soils differs from one to the other. In addition, the water content increases gradually with the rice husk rate in the different mixtures.
2.2.2. Production of Specimens
The mixtures obtained after mixing were placed in the moulds of dimension 100 × 100 × 10 mm3 for a maturation time of 0 days, 7 days and 14 days. The demoulding was done about one hour later. The samples were dried for 24 hours in the manufacturing room and then in the oven where the temperature was gradually increased from 35˚C to 60˚C for 24 hours to eliminate the presence of water in the pores of the adobes. The completely dried samples were sanded with green paper (Figure 2(c)) to make their surfaces flat and thus promote good contact with the heating element. Figure 2 shows the storage of the adobes during drying and the sanded adobes.
2.3. Experimental Setup and Principle of the Methods
The apparatus used in the experiments is shown in Figure 3, and is used to experimentally determine thermal conductivity, thermal effusivity, thermal capacitance and thermal diffusivity of different samples in accordance with standard NF EN 993-15 of 2005 [17] . This method is used by the Laboratory of Energetics and Theoretical and Applied Mechanics (LEMTA) at the National Center for Scientific Research of Nancy—University online (http://www.thermique55.com/principal/metrologiethermique.pdf) and referred to in the manuscript by [16] . This metrology is used by many whose work has been published as [3] [15] [18] [19] [20] . We have also been inspired by the ASMD5930-97 [21] standard related to the wire and hot plane method.
Principle of the Methods for Measuring the Thermal Properties of the Samples
The method of the asymmetrical finished hot plane with insulated back face was used to measure both thermal conductivity, thermal effusivity, thermal capacitance and thermal diffusivity of different samples. This method was developed to
![]()
![]()
Figure 2. (a) First drying phase of adobes, (b) sanded adobes, (c) green paper.
![]()
Figure 3. Asymmetric hot plane experimental setup.
measure the thermal properties of building materials, especially those made of earth, concrete, wood, plastic [16] .
The principle of this method is shown in Figure 4. an adobe sample is placed on a heating element with the same surface area (100 × 100 mm2), and a two-wire type K thermocouple with a diameter of less than 0.05 mm is inserted in the middle of the heating element on its underside, in contact with a block of polystyrene. On the upper side of the sample, another block of polystyrene is placed. The whole assembly is installed between two aluminum blocks, each 4 cm thick. When the device shown in Figure 4 is switched on, the generator voltage is set to 10.0 volts, corresponding to a current intensity of 0.25 Amperes. A flow of heat is diffused through the heating element and the evolution of the thermocouple temperature T(t) is recorded [3] [16] [18] [20] [22] . The presence of the thermocouple cannot generate thermal resistance, as it is in contact with the polystyrene, which is an insulator [16] .
According to the authors [13] [16] , the system is modeled on the assumption that heat transfer at the center of the heating element, and therefore of the sample,
![]()
Figure 4. Schematic of the asymmetric hot plane experimental setup in section [3] [16] [21] .
remains unidirectional (1D) for the duration of the experiment. The temperatures T(t) recorded under this assumption are processed using Excel software. The thermos-physical parameters are estimated from the complete model, taking the values of the pre-estimated thermal parameters as initial values. The temperatures T(t) recorded under this assumption are processed using Excel. These assumptions lead to Equations (1), (2) and (3) below. These equations were used by [13] [16] [20] during their work.
(1)
(2)
(3)
θ is the Laplace transform of the temperature rise T(t);
Φ01 is the Laplace transform of the heat flux density dissipated by the heating element to the adobe sample under test (upward);
Φ02 is the Laplace transform of the heat flux density dissipated by the heating element to the polystyrene placed at the bottom of the adobe sample;
Φ0 is the Laplace transform of the total heat flux density produced by the heating element;
f0 is the total heat flux density produced by the heating element;
Ch is the heat capacity of the heating element per unit area (
);
Rc is the contact resistance of the heating element and the adobe sample;
Φ1 is the Laplace transform of the heat flux density arriving at the top aluminium block;
Φ2 is the Laplace transform of the heat flux density arriving at the bottom aluminium block.
(4)
(5)
(6)
λ is the thermal conductivity of the adobe sample;
a is the thermal diffusivity of the adobe sample;
ρc is the thermal capacitance of the adobe sample;
E is the thermal effusivity of the adobe sample;
e is the thickness of the adobe sample;
λi, ai and ei denote the thermal conductivity, thermal diffusivity and thickness of the polystyrene, respectively.
The combination of Equations (1), (2), (3), (5), (6) gives:
(7)
The Levenberg-Marquardt algorithm [23] [24] integrated in a Matlab code allows to estimate the thermal parameter values that minimize the sum of the squared deviations
[25] between the experimental curves
and the theoretical curves evaluated by the inverse Laplace transform of Equation (7) [13] . The inverse Laplace transform is obtained using the Hoog algorithm [26] reported in the papers [13] [16] .
3. Results and Discussion
3.1. Residuals and Reduced Temperature Sensitivity Curves to Parameters
The estimation of the thermal effusivity and thermal conductivity of adobe samples is essentially based on the residual, which is the difference between the theoretical curve and the experimental curve, and on the reduced sensitivity curves [25] . This estimation is done on a time interval [t0, tmax] such that the residuals are centered around zero degrees, which validates the 1D model [27] [28] .
3.1.1. Residue Analysis
Figure 5 shows the experimental and model temperature curves for adobes reinforced with 0%, 1%, 1.5%, 2% and 2.5% rice husks, and the resulting residual curves. These adobes are produced from N’Djamena soil (NDJA) and Moundou soil (MOD). When the residual curve is centered on 0°C, there is no difference between Texp and Tmod, and they overlap. At this point, the thermal measurement validation condition is 100%. Meanwhile, the red color indicating Texp on the legend is not easily observable on the various curves in Figure 5. If it is,
it appears faintly in Figure 5(g). Analysis of the curves in Figure 5 shows that the residual curves are mostly centered around 0˚C. This means that the condition of thermal measurement and unidirectional heat transfer is satisfied. The Tmods of the samples are similar, as the variation in temperature measurement is similar. However, we note that the time required to estimate the thermal parameters is of the order of 300 and/or 350 seconds. The curves in Figure 5 are similar to those reported by [16] . The authors [27] [28] obtained similarly shaped residual curves by measuring the thermal properties of building materials.
3.1.2. Experimental and Model Temperature Curves with x (10) Residue Curves of NDJA and MOD Soils with 0%, 1%, 1.5%, 2%, 2.5% of 1.25 mm Rice Husks
Figure 6 shows two curves of reduced sensitivities of thermal effusivity (E), volumetric heat capacity (rhoCp), and temperature contact resistance (Rc). Reduced sensitivities have the advantage of allowing direct comparison of the relative influence of different parameters on temperature. For separate estimation of the parameters to be possible, the sensitivities of T(t) to each of the parameters must be decorrelated. Analysis of the sensitivity curves of the heated face of the samples shows that:
The sensitivity of T(t) to contact resistance at the probe/sample interface is high for our specimens at the beginning of the thermal measurement but varies slightly after 100 s. It is therefore not possible to estimate this parameter on the portion of the T(t) curve corresponding to t greater than 100 s;
The sensitivity of E is strong and uncorrelated with the sensitivities of rhoCp and Rc. Therefore, it is possible to estimate E and rhoCp separately from the experimental T(t) curve (Figure 6). On the other hand, the sensitivity curve reduced
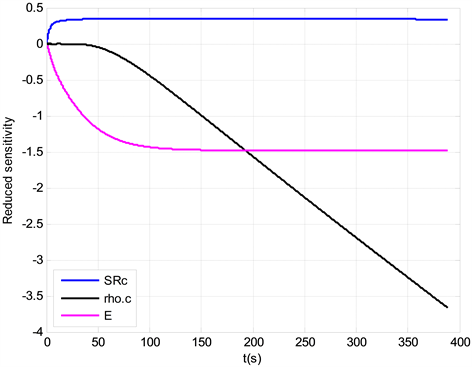
(a) NDJA with 0% rice husks.
![]()
(b) MOD with 0% rice husks.
to E varies very little from 300 s, while the sensitivity curve reduced to rhoCp temperature varies from 50 s. From the above the estimation of the parameters will therefore only be accurate in the interval from 50s to 300s where the curves stabilize.
3.2. Thermal Conductivity
Figure 7 shows the variation in thermal conductivity of the MOD and NDJA soils as a function of the rice husk content into the adobes and the time it takes for the adobe pastes to be molded. Analysis of the different curves for these two rice husk-reinforced soils shows that the thermal conductivity of the samples decreases as the portion of rice husks incorporated into the adobes increases. This incorporation induces internal pores in the clay, which favors the decrease in conductivity. Similar results have been obtained by others researchers [9] [22] [29] [30] [31] who performed thermal conductivity measurements of raw earth composites and plant fibers. The thermal conductivity values of bricks without theaddition of rice husks range from 0.912 to 0.657 W∙m−1∙K−1 depending on the maturation time for MOD soil and from 1.118 to 0.894 W∙m−1∙K−1 for NDJA soil depending on the maturation days. These values are comparable to those obtained by Babé et al. [22] , Mathioro et al. [27] and Ouédraogo [31] .
Further analysis of the results in Figure 7 shows that the maturation of the composites is favourable to thermal insulation. Because the longer the adobe pastes take to be moulded, the lower the thermal conductivity value. However, for the MOD soil the thermal conductivity value is lower at zero days of maturation for the rice husk content of 2.5%. Bobet [9] had found during his work when he incorporated 5% - 20% peanut hulls into a clay soil up to 3 days of
![]()
![]()
Figure 7. Variation in thermal conductivity of MOD and NDJA soils as a function of rice husk content and adobe paste maturation time.
ripening the adobes show a slightly higher thermal conductivity than that obtained at 5 days of ripening. This contrast in results can be explained by the low content of rice husks which could not release sufficient amount of macromolecules such as cellulose which is favorable to conductivity on the one hand and/or the reduction of the number of macromolecules in the composites with time under the interaction of the clay matrix on the other hand. This can confirm the result of Bobet [9] at 5 days of maturation.
Table 3 shows the percentage improvement in thermal comfort of the studied adobes. The improvement decreases with maturation time for the MOD soil while it is disparate for the NDJA where the maximum percentage improvement is at day 7 of maturation. Overall, the incorporation of rice husks in both clay soils contributes to the improvement of thermal comfort in buildings constructed with these composite materials.
3.3. Thermal Effusivity
The thermal effusivity of a material is its ability to exchange thermal energy with its environment by conduction as a function of time. The thermal effusivity of adobe samples is measured by the same experimental device as the conductivity as shown in Figure 8. Like the thermal conductivity, it decreases as a function of the rice husk content in the clay matrix. The analysis of the curves in Figure 8 shows that the effusivity of the adobes without or with the addition of rice husks decreases with the maturation time and the percentage of rice husks for the NDJA soil. For the MOD soil this variation does not follow the same logic for the samples without addition. The effusivity value is 1135.321 J∙m−2∙K−1∙s−1/2 for the adobe (MOD) without husk addition on day 7 and is a little higher than the others. This value is far removed from the others and does not follow the logic of effusivity decay of the adobes containing the rice husks. This decay of thermal effusivity is a function of the fiber content incorporated in the clay. Similar results were obtained by Mathioro F. et al. [27] . Table 4 shows that for a rate of 2.5% of incorporated husks, the effusivity drops by 32%, 24%, 19% for MOD soil and 11%, 13%, 13% for NDJA soil depending on the maturation time of adobe pastes. For the NDJA the effusivity drop rates of the 7th and 14th day of maturation are identical. These results show that the effusivity drop rate also depends on the nature of the soil as the case of thermal conductivity. We note that the thermal effusivity and thermal conductivity of soils without addition are high: which confirms the results obtained by Lamrani M. et al. [13] .
![]()
Table 3. Thermal conductivity improvement rates for 2.5% rice husks added to MOD and NDJA soils.
![]()
Table 4. Rate of fall in thermal effusivity for a 2.5% addition of rice husks to MOD and NDJA soils.
![]()
![]()
Figure 8. Variation in thermal effusivity of MOD and NDJA soils as a function of rice husk content and adobe paste maturation time.
Table 4 shows the decrease of thermal characteristics of the studied adobes. The improvement of the decrease with the maturation time for the MOD soil while it is reversed for the NDJA but with a decrease not as noticeable as the case of the MOD soil. Overall, the incorporation of rice husks in the two clay soils contributes to the decrease of thermal effusivity in the studied adobes.
3.4. Thermal Volume Capacity and Thermal Diffusivity
The heat capacity and thermal diffusivity of the adobe samples made from the two soils (MOD and NDJA) were measured using the same experimental procedure as for conductivity and effusivity measurements. Their changes as a function of rice husk content are shown in Figure 9 and Figure 10, respectively. They also depend on the time taken by the adobe pastes before molding. The longer the pastes last, the lower the values of the thermal volume capacities of
these soils. We observe the opposite phenomenon for the thermal diffusivity. Analysis of the curves in Figure 9 shows that in 14 days of maturation of the pastes, the MOD volume capacity decreases to 1.10 × 10+6 J∙m−3∙K−1 for 2.5% of rice husks. However, this value is still slightly higher than that of the NDJA soil, which is 9.00 × 10+5 J∙m−3∙K−1 for the same rice husk rate. The thermal diffusivity values obtained during this measurement (2.47 × 10−7 to 4.21 × 10−7 J∙m−2∙K−1∙s−1/2) are of the same order of magnitude as those found by Lamrani et al. [13] . The results obtained by Ngono et al. [19] when measuring the heat capacity and thermal diffusivity of three types of tropical wood as a function of water content show that these thermal properties evolve in a comparable way to those of the adobes studied in relation to rice husks.
![]()
![]()
Figure 9. Variation in heat capacity of MOD and NDJA soils as a function of rice husk content and adobe paste maturation time.
![]()
![]()
Figure 10. Changes in thermal diffusivity of MOD and NDJA soils as a function of rice husk content and number of days of adobe paste maturation.
Figure 11 and Figure 12 show the percent lowering of the heat volume capacity and the rate of increase of the thermal diffusivity of the studied soils, respectively. It can be seen that the NDJA soil shows a high rate of lowering (Figure 11) while a lower rate of increase occurs for the MOD soil. This difference in the behavior of the MOD soil observed in Figure 11 and Figure 12 could be explained by the results of analysis of these two soils in the civil engineering laboratory at the National Higher School of Public Works of N’Djamena. The geotechnical study of these two soils conducted in the engineering laboratory showed that the MOD soil is slightly more plastic (plasticity limit equal to 24) than the NDJA soil (plasticity limit equal to 13). These two soils have respectively for Skempton activity value 0.91 and 1.03 allowing to conclude that the
![]()
Figure 11. Rate of decline in heat capacity of MOD and NDJA soils between 0% and 2.5% rice husk 1.25 mm adobes as a function of maturation time.
![]()
Figure 12. Rate of increase in thermal diffusivity of MOD and NDJA soils between 0% and 2.5% 1.25 mm rice husks as a function of maturation time.
MOD soil contains illite clay minerals while the NDJA soil contains smectite and illite clay minerals. The illite has a low cation exchange capacity while smectites have a high cation 4 exchange capacity because of the size of their interfoliar space [9] . In addition, the characteristics of clays are mainly under the control of their specific surface area [29] . MOD soil and NDJA have 36.93 m2/g and 58.58 m2/g specific surface area, respectively.
4. Conclusions
The main objective of this study was to measure the thermophysical characteristics of soil blocks stabilized with rice husks at different proportions. The measurements were carried out using the asymmetrical hot plane method. The different values of the thermal parameters obtained during the measurements show that the incorporation of rice husks into the clay matrix induces a drop in thermal conductivity, volumetric heat capacity and thermal effusivity as a function of the rice husk ratio. The results show that the maturation time of rice husk- stabilized block pastes is also conducive to a reduction in these thermal parameters. The results of this study could be used to improve thermal comfort in building construction.
Our future perspectives, we would like to model and simulate the thermal behavior of these stabilized blocks to better appreciate the experimental results obtained.
Acknowledgements
The authors sincerely thank the Director General of National High School of Public Works of N’Djamena, Chad, for providing us with the necessary means to access the energy laboratory of National Higher Polytechnic School of Yaoundé (ENSPY) of Yaoundé, Cameroon.