Involvement of Dectin-2 in the Innate Inflammatory Response Triggered by Influenza Virus Hemagglutinin ()
1. Introduction
Influenza is a well-known viral infection, prevalent worldwide, that causes upper respiratory tract symptoms and pneumonia in people of all ages [1] . Previous report estimated that approximately 300,000 to 600,000 people in the world annually die from influenza-associated respiratory disorders [2] . In addition, influenza is not only a physical burden but also an economic cost, with estimates showing that the U.S. spends an average of $11.2 billion to the healthcare society per year [3] . Most people only exhibit cold-like symptoms, such as high fever and muscle aches. However, patients with underlying diseases related to severe influenza are more susceptible to severe disease; this can lead lethal forms of secondary bacterial pneumonia mainly caused by Streptococcus pneumoniae [4] . Acute respiratory distress syndrome (ARDS), characterized by severe respiratory failure due to pulmonary edema, capillary leakage, and hypoxemia, was increasingly prevalent in the 2009 A/H1N1pdm09 pandemic [5] . Quadrivalent inactivated split vaccine has been used in Japan to prevent the aggravation of influenza virus (IFV) infection as mentioned above, but there are several problems with this vaccine strategy. The current influenza vaccine is less effective for people with no history of influenza. Furthermore, its preventive effect varies depending on the type of prevalent strain or host age [6] . In addition, split vaccines, which are mainly composed of hemagglutinin (HA) expressed on the membrane surface of IFV, are not sufficiently effective, especially in infants, due to their low ability to induce innate immune responses [7] . To overcome these challenges and achieve more effective prevention, it is important to address the mechanism of the host immune response against IFV infection.
The host defense against pathogenic microorganisms is initiated by the recognition of microbial molecules by antigen-presenting cells (APCs), namely dendritic cells (DCs) and macrophages. These molecules are generally called pathogen-associated molecular patterns (PAMPs) and include a wide variety of peptides, nucleic acids, and polysaccharides. The characteristic receptors called pattern recognition receptors (PRRs), including Toll-like receptors (TLRs), C-type lectin receptors (CLRs), and Nod-like receptors (NLRs), are known to activate intracellular signaling pathways by recognizing PAMPs. This is followed by the initiation of immune responses such as inflammatory cytokine production and co-stimulatory molecule expression [8] . Furthermore, the recognition of viral RNA by TLR3 and TLR7 triggers the primary immune response in the host defense against IFV infection and promotes antiviral responses by inducing the production of type I interferons (IFNs) [9] . Involvement of CLR-mediated host immune responses has also been reported. One notable report showed that influenza pneumonia was reduced when caspase recruitment domain family member 9 (CARD9), an essential signaling molecule downstream of CLRs, was absent [10] . In addition, the analysis focused on Clec5a; a murine model of influenza pneumonia showed that the inflammatory response in the lungs was reduced in Clec5a gene-deficient mice [11] . These reports suggested that CLRs may be involved in suppressing severe inflammation in infected lungs, but the detailed molecular mechanisms regulating this viral pneumonia are not clear.
Vaccines provide an important role in preventing infectious diseases by equipping the body in advance with neutralizing antibodies against microorganisms. To strengthen the effectiveness of vaccines, inorganic substances such as squalene or aluminum hydroxide are often used and are the most representative adjuvants in IFV infection [12] . In recent years, adjuvants targeting pathogen-specific molecules such as nucleic acids and lipopolysaccharides (LPS), sensed by innate immune receptors, including intracellular or extracellular PRRs, have been vigorously examined [13] . For example, CpG 1018 effectively equips neutralizing antibodies against a new coronavirus (SARS-CoV-2) and is expected to be applied for IFV infection [14] . In addition, trehalose dibehenate (TDB), a synthetic analog of glycolipid trehalose dimycolate (TDM) in the cell wall of Mycobacterium tuberculosis, is known as an adjuvant that targets CLRs in tuberculosis [15] . In the control of IFV infection, it is an academical importance in the anti-viral defense strategy to elucidate the host immune response mediated by IFV surface polysaccharides via CLRs because the interactions between APCs and viral surface antigens are critical for the initiation of rapid inflammation, rather than the previously reported innate response triggered by intracellular IFV nucleic acids via TLRs. Therefore, to investigate clinically applicable adjuvants based on CLRs is an important area for developing novel vaccine strategies to explain the host immune response triggered by CLRs against IFV infection.
We have previously reported that Dectin-2, a CLR recognizing high mannose polysaccharide, is involved in the host immune responses initiated by IFV HA [16] . In the present study, we carried out further detailed analysis on the involvement of Dectin-2 in the inflammatory response induced by various types of HA. The stimulation with HA treated with periodic acid or endoglycosidase resulted in a significantly reduced inflammatory cytokine production from bone marrow-derived dendritic cells (BM-DCs) compared with untreated HA stimulation. Furthermore, inflammatory cytokine secretion by peritoneal macrophages was significantly decreased in Dectin-2 knockout (KO) mice. In contrast, IFN-α production by CD11c+Siglec-H+PDCA-1+ plasmacytoid DCs (pDCs) was significantly increased in Dectin-2 KO mice compared with wild-type mice. These results suggested that Dectin-2 induced innate immune responses via mannose polysaccharide on HA, although other mechanisms may function in the antiviral response induced by the type I IFN axis.
2. Materials and Methods
2.1. Ethical Statement
This study was performed in strict accordance with the Fundamental Guidelines for Proper Conduct of Animal Experiment and Related Activities in Academic Research Institutions under the jurisdiction of the Ministry of Education, Culture, Sports, Science and Technology in Japan, 2006. All experimental procedures involving animals followed the Regulation for Animal Experiments at Niigata University and Tohoku University and were approved by the Institutional Animal Care and Use Committee of Niigata University and the ethics committee of Tohoku University (approval numbers SA00615, SA00949, SD01280, and SD01709). All experiments were performed under anesthesia to minimize animal distress.
2.2. Mice
Dectin-2 KO mice were established as described previously [17] , and backcrossed to C57BL/6J mice for more than eight generations. Wild-type (WT) C57BL/6J mice purchased from the Charles River Laboratories Japan, Inc. (Yokohama, Japan) were used as controls. Six- to eight-week-old male mice were used in the experiments. All mice were bred under specific pathogen-free conditions at the Department of Comparative and Experimental Medicine, Niigata University, Japan.
2.3. Reagents
HAs were derived from IFVs, including A/Victoria/1/2020(IVR-217)(H1N1) pdm09, A/Tasmania/503/2020(IVR-221)(H3N2), B/Victoria/705/2018(BVR-11) (Victoria lineage), and B/Phuket/3073/2013 (Yamagata lineage) and quadrivalent seasonal influenza HA vaccine were purchased from Denka Seiken Co., Ltd. (Niigata, Japan), and were prepared according to the manufacturer’s protocol for the seasonal influenza HA vaccine in Japan. Briefly, viruses were cultured in embryonated eggs and concentrated by density-gradient centrifugation. After purification, the HA fractions from the virions were extracted and stabilized. All HAs were dialyzed in RPMI 1640 medium three times and stored at −80˚C until use. LPS was purchased from Sigma Aldrich (St. Louis, MO, USA). Immunostimulatory oligodeoxynucleotide CpG 1826, which is a TLR9 agonist, 20 nucleotides in length, was synthesized by Hokkaido System Science (Sapporo, Japan).
2.4. Preparation and Culture of DCs
Bone marrow cells from C57BL/6J mice or Dectin-2 KO mice were cultured at 2 × 105/mL in 10 mL of RPMI 1640 medium supplemented with 10% fetal bovine serum (FBS), 100 U/mL penicillin G, 100 µg/mL streptomycin, and 50 µM 2-mercaptoethanol containing 20 ng/mL murine granulocyte-macrophage colony-stimulating factor (GM-CSF; Fujifilm Wako Pure Chemical Corporation, Ltd., Osaka, Japan). On day 3, another 10 mL of the same complete medium was added, and on day 6, a half-volume of medium was removed and replaced by fresh culture medium. On day 8, non-adherent cells were collected and used as conventional BM-DCs. In another experiment, bone marrow cells from C57BL/6J mice or Dectin-2 KO mice were cultured at 1 × 106/mL in 10 mL of complete medium with 100 μg/mL FMS-like tyrosine kinase 3 ligand (Flt-3L; Fujifilm Wako Pure Chemical Corporation, Ltd., Osaka, Japan). On day 3, another 10 mL of the same complete medium and Flt-3L were added. On day 5, floating cells were collected and used as pDCs. The obtained DCs were cultured with various doses of HA, Furfurman (InVivoGen, San Diego, CA, USA), CpG 1826, or LPS for 24 h at 37˚C under 5% CO2 conditions.
2.5. Preparation of Peritoneal Macrophages
DifcoTM Fluid Thioglycolate Medium (Becton Dickinson, Franklin Lakes, NJ, USA) was dissolved in sterilized water with heating to a concentration of 3% and then autoclaved. After several months of standing in the dark, this medium (1.5 mL per mouse) was administered intraperitoneally. At 4 days post injection, the intraperitoneal cavity was washed with RPMI 1640 medium supplemented with 10% FBS and 10 mM HEPES, and intraperitoneal cells were harvested. The collected cells were seeded into sterile glass Petri dishes and incubated in a CO2 incubator at 37˚C for 2 h. Cells floating in the supernatant were removed, and only adherent cells were collected and used as peritoneal macrophages. The obtained peritoneal macrophages were cultured with the indicated HA, Furfurman, or LPS doses for 24 h at 37˚C under 5% CO2 conditions.
2.6. Treatment of Endoglycosidase
Each type of HA was treated with PNGase F, O-glycosidase, β-N-acetylglucosaminidase S, α1-3,4,6 galactosidase, β1-3,4 galactosidase, or α1-2,3,6 mannosidase (New England Biolabs, Ipswich, MA, USA) according to the manufacturer’s instructions. The untreated or enzyme-treated HA was then added into 96-well culture plates at the indicated doses and plates were incubated overnight at 4˚C. After removing the supernatant, the wells were washed with sterilized phosphate-buffered saline, and then BM-DCs derived from WT mice were added to each well and incubated for 24 h at 37˚C under 5% CO2 conditions.
2.7. Cytokine Assay
Interleukin (IL)-12p40 and IL-6 concentrations in culture supernatants were measured using an enzyme-linked immunosorbent assay (ELISA) kit (BioLegend, San Diego, CA, USA). The IFN-α concentration was also detected using the VeriKine-HS Mouse Interferon Alpha All Subtype ELISA Kit (PBL Assay Science, Piscataway, NJ, USA). The sensitivity was 4 pg/mL for IL-12p40, 2 pg/mL for IL-6, and 2.38 pg/mL for IFN-α.
2.8. NFAT-Green Fluorescent Protein (GFP) Reporter Assay
Nuclear factor of activated T cells (NFAT)-GFP reporter cells expressing the indicated CLRs were prepared as previously described [18] . Briefly, T cell hybridoma 2B4 was transfected with the NFAT-GFP construct, which was prepared by fusing three tandem NFAT-binding sites with enhanced GFP cDNA. NFAT-GFP transfected cells were stimulated with anti-TCR-β antibody and GFP-positive cells were collected. This cell line was transfected with the Dectin-2 gene, together with the FcRγ gene, and the same cell line that lacked the receptor gene was used as a control. In addition, reporter cells transfected with a Dectin-2 gene in which the amino acid sequence of the carbohydrate-binding domain of the Dectin-2 was mutated from EPN (Glu-Pro-Asn) to QPD (Gln-Pro-Asp), were used [19] [20] . These reporter cells were stimulated for 20 - 24 h with various types of HA or seasonal influenza vaccine, and GFP expression on CD3+ cells was analyzed using a flow cytometer. The vertical axis of the histogram shows the number of cells as a real number, and the horizontal axis represents the GFP fluorescence intensity as a logarithm.
2.9. Statistical Analysis
Each experiment was repeated twice with similar results. Data were expressed as the mean ± standard deviation (SD). Differences between groups were examined for statistical significance using Welch’s t-test. A P-value less than 0.05 was considered to be significant.
3. Results
3.1. Effect of Deglycosylation against HA on Cytokine Production by BM-DCs
To investigate the involvement of high mannose polysaccharide modified on HA in the initiation of inflammatory responses, periodate treatment of HA was performed, and the effect on cytokine production by DCs derived from WT mice and Dectin-2 KO mice was analyzed. Mannose polysaccharide is oxidized by periodate, yielding formic acid and aldehydes. Therefore, BM-DCs from WT mice were stimulated with each HA treated with or without periodate for 24 h, and IL-12p40 production in the supernatant was evaluated. As shown in Figure 1(A), IL-12p40 production was significantly reduced by the stimulation with periodate-treated HA compared with untreated HA. In contrast, no effect of periodate treatment was observed after stimulation with CpG 1826, an oligonucleotide. Polysaccharide modifications are found on the surface of representative viral antigens such as HA and neuraminidase. Only N-linked glycans, classified into types based on terminal glycan branching structure including predominantly high mannose glycans, complex glycans, and hybrid glycans, are present on the HA surface [21] [22] . Therefore, to elucidate the involvement of mannose polysaccharide from another perspective, each HA was treated with or without various endoglycosidases, and the IL-12p40 concentration in the culture supernatant
![]()
Figure 1. Effect of deglycosylation of hemagglutinin (HA) on cytokine production by bone marrow-derived dendritic cells (BM-DCs). (A) Each type of HA (10 μg/mL) and CpG 1826 (1 μg/mL) was incubated with periodic acid (1 mM) at 4˚C in the dark for 1 h. BM-DCs from wild-type (WT) mice were co-cultured with the treated or untreated HA and CpG 1826 for 24 h. Interleukin (IL)-12p40 concentration in the culture supernatants was measured using enzyme-linked immunosorbent assay (ELISA). (B), (C) Here, 10 μg of each type of HA were treated with peptide: N-glycosidase F (PNGase F; 20,000 U), O-glycosidase (80,000 U), β-N-acetylglucosaminidase S (40 U), α1-3,4,6 galactosidase (80 U), β1-3,4 galactosidase (80 U), or α1-2,3,6 mannosidase (20 U) at 37˚C for 24 h. These treated or untreated HAs were adjusted to a 10 μg/mL concentration, then added to 96-well culture plates and incubated overnight at 4˚C. After incubation, the supernatant was removed and the plates were washed with sterilized phosphate-buffered saline (PBS). Then BM-DCs were added to the plates and incubated at 37˚C for 24 h. The IL-12p40 concentration in the culture supernatants was measured by ELISA. Each column represents the mean ± standard deviation (SD) of triplicate cultures. Statistical analysis compared the untreated (shown as (-)) and enzyme-treated samples for each HA. Experiments were repeated twice with similar results. medium, RPMI 1640 medium supplemented with 10% fetal bovine serum; (-), untreated sample; PNG, PNGase F; O-Gly, O-glycosidase; GlcNAc; β-N-acetylglucosaminidase S, β-Gal; β1-3,4 galactosidase, α-Gal; α1-3,4,6 galactosidase, α-Man; α1-2,3,6 mannosidase; *P < 0.05.
was detected upon BM-DC stimulation with these HA. As shown in Figure 1(B), IL-12p40 production was significantly reduced in cells stimulated with PNGase F-treated HA compared with the untreated group; this enzyme cleaves N-linked glycans. In contrast, HA treatment with O-glycosidase, an enzyme that cleaves O-linked glycans, did not affect IL-12p40 production by BM-DCs in both groups. Furthermore, A/H3N2 subtype and B/Victoria lineage HA treated with α-mannosidase resulted in a significant IL-12p40 reduction in BM-DCs compared with the untreated group (Figure 1(C)). These results indicated that N-linked glycan, especially mannose polysaccharide, is involved in the initiation of the inflammatory response by DCs.
3.2. Effects of Amino Acid Mutation in the Carbohydrate-Binding Domain of Dectin-2 on Binding to HA
Our previous report showed that Dectin-2 binds directly to HA, particularly A/H3N2 subtype HA [16] . Next, we analyzed whether amino acid mutation of the carbohydrate-binding site of Dectin-2 altered its ability to bind to HA. In this experiment, GFP expression by reporter cells transfected with the canonical Dectin-2 gene (shown as Dectin-2-EPN) or with Dectin-2 with mutated amino acids in the carbohydrate recognition domain (shown as Dectin-2-QPD) was examined by flow cytometry after stimulation with various types of HA. As shown in Figure 2, GFP expression in Dectin-2-EPN cells was approximately 3% - 12% following HA stimulation, except for A/H1N1pdm09 subtype HA. In contrast, expression in Dectin-2-QPD-expressing cells was considerably reduced compared with that in Dectin-2-EPN cells, especially after stimulation with A/H3N2 subtype HA. After stimulation with A/H1N1pdm09 subtype HA, GFP expression in Dectin-2-QPD cells was comparable with that in Dectin-2-EPN cells, although slight GFP expression was observed after co-culture with Dectin-2-EPN cells. Binding to Furfurman, a ligand specific for Dectin-2, resulted in 61% GFP expression in Dectin-2-EPN cells, whereas this expression was markedly reduced to approximately 2% in Dectin-2-QPD cells. These results suggested that HA is involved in binding to Dectin-2, especially A/H3N2 subtype HA.
3.3. Cytokine Production by Peritoneal Macrophages upon Stimulation with HA
Our experiments focused on DCs, which are the APCs most pivotal in initiating the host immune responses against the primary invasion of microorganisms. In addition, macrophages, another representative APC, are essential for effectively eliminating viruses through viral recognition and phagocytosis [23] . Therefore, to investigate the involvement of Dectin-2 in activation of macrophages by HA, peritoneal macrophages, induced by intraperitoneal administration with thioglycolate medium against WT mice and Dectin-2 KO mice, were stimulated with various types of HA. The production of proinflammatory cytokines in WT mice
![]()
Figure 2. Effects of amino acid mutation in the carbohydrate-binding domain of Dectin-2 on binding to hemagglutinin (HA). The reporter cells expressing murine Dectin-2, or Dectin-2 in which the amino acid sequence of the carbohydrate-binding domain was changed from EPN (Glu-Pro-Asn) to QPD (Gln-Pro-Asp), were co-cultured with various types of HA (10 μg/mL) for 20 - 24 h, and green fluorescent protein (GFP) expression was analyzed using a flow cytometer. Cells not expressing these receptors were used as the control. As the positive control, Furfurman, which is a Dectin-2 ligand, was used. Representative data from independent experiments are shown. Experiments were repeated twice with similar results.
and Dectin-2 KO mice was then compared. As shown in Figure 3, IL-12p40 and IL-6 production, upon stimulation with each type of HA, was significantly reduced in Dectin-2 KO mice compared with WT mice. These results indicated that Dectin-2 is involved in producing a proinflammatory cytokine response in macrophages stimulated with HA, suggesting that Dectin-2 may also contribute to viral clearance or the promotion of the host inflammatory response via the activation of macrophages.
3.4. Contribution of Type I Interferon Production by HA-Stimulated Plasmacytoid DCs
Type I IFNs, including IFN-α and IFN-β, are the cytokines most essential in the antivirus response. Type I IFNs are mainly secreted by pDCs and act on both infected and uninfected cells through the type I IFN receptor (IFNAR1), resulting in suppressing virus replication by activating various intracellular transcription factors in virus invasion [24] . To investigate the role of Dectin-2 in type I IFN production induced by HA, pDCs differentiated by Flt-3L were obtained from
![]()
Figure 3. Cytokine production by peritoneal macrophages upon stimulation with hemagglutinin (HA). WT or Dectin-2 KO mice were injected intraperitoneally with 3% thioglycolate medium. After 4 days, the intraperitoneal cavity was washed with 10% fetal bovine serum (FBS)/RPMI 1640 medium supplemented with 10 mM HEPES. These harvested intraperitoneal cells were incubated in sterile glass Petri dishes for 2 h and adherent cells were used as peritoneal macrophages. Collected peritoneal macrophages were co-cultured with the indicated dose of various types of HA, Furfurman (100 μg/mL), or lipopolysaccharide (LPS; 1 ng/mL) for 24 h. IL-12p40 and IL-6 concentrations in the culture supernatants were measured by enzyme-linked immunosorbent assay (ELISA). Each column represents the mean ± standard deviation (SD) of triplicate cultures. Experiments were repeated twice with similar results. *P < 0.05.
WT mice and Dectin-2 KO mice and co-cultured with various types of HA, and then the IFN-α concentration in the culture supernatant was measured. The pDCs, defined as CD11c+Siglec-H+PDCA-1+ cells, were confirmed to be approximately 21% of the CD11c+ cells using flow-cytometric analysis (Supplemental Figure 1). As shown in Figure 4(A), IFN-α production was significantly increased in Dectin-2 KO mice compared with WT mice upon stimulation with A/H1N1pdm09 subtype, A/H3N2 subtype, or B/Victoria lineage HA. However, there was no significant difference in IFN-α concentration following stimulation with B/Yamagata lineage HA between WT mice and Dectin-2 KO mice. In addition, IL-12p40 levels in the same culture supernatant were significantly lower in Dectin-2 KO mice compared with WT mice after stimulation with all types of HA (Figure 4(B)). This result was consistent with our previously published data [16] . These results suggested that the type I IFN response induced by HA was enhanced under Dectin-2 deficiency.
![]()
Figure 4. Type I interferon production by hemagglutinin (HA)-stimulated plasmacytoid dendritic cells (pDCs). Bone marrow cells from wild-type (WT) mice and Dectin-2 knockout (KO) mice were co-cultured with FMS-like tyrosine kinase 3 ligand (Flt-3L; 100 μg/mL) for 5 days and non-adherent cells were used as pDCs. Prepared pDCs were co-cultured with indicated doses of various types of HA (10 μg/mL) and CpG 1826 (1 μg/mL) for 24 h. After stimulation, interferon (IFN)-α (A) and interleukin (IL)-12p40 (B) concentrations in the culture supernatants were measured using ELISA. Each column represents the mean ± standard deviation (SD) of triplicate cultures. Experiments were repeated twice with similar results. *P < 0.05.
3.5. Immune Response by BM-DCs upon Stimulation with Quadrivalent Seasonal HA Vaccine
As we determined the inflammatory response upon stimulation with each type of HA alone, we finally investigated the involvement of Dectin-2 in the immune response triggered by stimulation with quadrivalent seasonal HA vaccine, which is a mixture of the four types of HA. Dose-dependent IL-12p40 production by BM-DCs was observed in WT mice. In contrast, this production was not detected in Dectin-2 KO mice following stimulation with the vaccine (Figure 5(A)). Because binding to each type of HA had been examined using Dectin-2 gene-transduced reporter cells (Figure 2), the interaction between Dectin-2 and seasonal influenza vaccine was also evaluated. As shown in Figure 5(B), approximately 2.8% GFP expression in Dectin-2-EPN cells was detected upon stimulation with seasonal influenza vaccine. In contrast the expression in Dectin-2-QPD cells was reduced to approximately 47% that of Dectin-2-EPN cells. These data suggested that Dectin-2 was also involved in the immune response triggered by HA when the four types of HA were mixed.
![]()
![]()
Figure 5. Dectin-2-mediated cytokine production and binding upon stimulation with quadrivalent seasonal influenza vaccine. (A) Bone marrow-derived dendritic cells (BM-DCs) from wild-type (WT) or Dectin-2 knockout (KO) mice were co-cultured with quadrivalent seasonal influenza vaccine (1 or 3 μg/mL), Furfurman (100 μg/mL), or lipopolysaccharide (LPS; 1 ng/mL) for 24 h. Interleukin (IL)-12p40 concentration in the culture supernatants was measured by enzyme-linked immunosorbent assay (ELISA). Each column represents the mean ± standard deviation (SD) of triplicate cultures. *P < 0.05. (B) The reporter cells expressing murine Dectin-2, or Dectin-2 in which the amino acid sequence of the carbohydrate-binding domain was replaced from EPN (Glu-Pro-Asn) to QPD (Gln-Pro-Asp) were co-cultured with quadrivalent seasonal influenza vaccine (3 μg/mL) for 20 - 24 h, and green fluorescent protein (GFP) expression was analyzed using a flow cytometer. The cells without expression of these receptors were used as control. As positive control, Furfurman, a Dectin-2 ligand, was used. Representative data from independent experiments are shown. Each experiment was repeated twice with similar results.
4. Discussion
For IFV to enter host cells, HA needs to bind sialic acid, abundant on the plasma membrane of epithelial cells. Conversely, sialic acid-independent recognition and internalization of IFV-derived HA by APCs is initiated by PRRs expressed on the surface of DCs and macrophages, and these responses are the first contact with the host immune cells upon entry into the lung. Therefore, we focused on glycosylated HA and analyzed the interaction of various types of HA with Dectin-2, also known as a PRR, involved in the recognition of microbial high mannose polysaccharides. Previous reports have shown that DC-specific intracellular adhesion molecule 3 grabbing non-integrin (DC-SIGN) supports the intracellular invasion and replication of IFV particles, although it is not clear which components of IFV are sensed by DC-SIGN [25] [26] . HA contains various types of polysaccharides, including mannose, galactose, N-acetyl-galactosamine, and fucose, which constitute N-linked glycans classified into three types: high mannose type, hybrid type, and complex type [27] . In the present experiment, IL-12p40 secretion by BM-DCs was significantly reduced by treatment of HA with periodate or PNGase F when compared with the untreated group (Figure 1(B)). Analysis of each endoglycosidase showed that α-mannosidase treatment significantly reduced proinflammatory cytokine production by BM-DCs stimulated with HAs from the A/H3N2 subtype and B/Victoria lineage, but not A/H1N1pdm09 subtype or B/Yamagata lineage HAs (Figure 1(C)). These data suggested that A/H3N2 subtype and B/Victoria lineage HAs predominantly possess high mannose type N-linked glycans, whereas the A/H1N1pdm09 subtype and B/Yamagata lineage contain mainly hybrid type or complex type N-linked glycans. Based on a series of our data, HA from the A/H3N2 subtype is particularly reactive with Dectin-2 and likely contains high-mannose polysaccharide, a potential adjuvant candidate against Dectin-2. Since it has been shown the possibility that Dectin-2 functions as a novel adjuvant-recognition receptor, further detailed molecular structural analysis of glycans recognized by Dectin-2 may be needed.
Binding of various HAs to reporter cells transfected with Dectin-2 revealed that GFP expression was highest upon stimulation with A/H3N2 subtype HA, and markedly reduced by amino acid mutation in the carbohydrate recognition site of Dectin-2 (Figure 2). This expression was also observed upon stimulation with quadrivalent influenza vaccine, but the proportion of positive cells was approximately 3% (Figure 5(B)). Of the four types of HAs, Dectin-2 binds most strongly to the A/H3N2 subtype, while its binding to the other HAs is weak, so they may cancel each other out in vaccine stimulation, reducing the proportion of GFP-positive cells. Previous reports have shown that the glycosylation site at the top of the head of the HA1 subunit from the A/H1N1 or A/H3N2 subtype has undergone structural changes during viral evolution, and the frequency of glycosylation at above site is gradually increasing [28] [29] . Furthermore, N-linked glycans in the globular region of contemporary seasonal IFVs, especially HA from the A/H3N2 subtype, are more abundant than those of other strains [21] [30] . Reverse genetic analysis using A/H1N1 subtype HA showed that the addition of N-linked glycan to HA affected susceptibility to surfactant protein D (SP-D), and the administration of glycan-deleted HA to mice led to a reduced ability to neutralize IFV in the lung and caused detrimental pathogenesis [31] . As the high abundance of glycans on the heads of HA can easily interact with DCs via CLRs in the physiological environment, a great contribution of Dectin-2 is expected to initiate the effective immune responses mediated by HA. Although the glycosylation of HA has been reported to modify T cell-mediated humoral response in vaccination or infection [29] , Dectin-2 is suggested to transduce strong signals into innate immune response via high-mannose polysaccharide and promote humoral immune response.
Inflammatory cytokines secreted by immune cells are pivotal molecules in forming networks by bridging the innate and adaptive immune responses during IFV infection. Intranasal administration of IL-12, which activates natural killer cells in the innate phase of the immune response, to mice early in IFV infection reduces the viral load in bronchoalveolar lavage fluid and improves the clinical course [32] . In addition, intranasal infection in mice with mouse-adapted A/PR8/34 strain (H1N1) after the removal of alveolar macrophages settled in the lungs led to an increase in viral dose and the severe state, with decreased SP-D and life-threatening edema in infected lungs [33] [34] . In the present analysis, IL-12p40 and IL-6 production from peritoneal macrophages stimulated with HA was significantly decreased in Dectin-2 KO mice (Figure 3). These results indicate that Dectin-2 may be closely involved in viral phagocytosis by alveolar macrophages and elimination by the mobilization of innate lymphoid cells (ILCs) during IFV infection. Conversely, in humans, proinflammatory cytokines such as IL-6 and IFN-γ in serum were increased significantly in patients who developed pneumonia during IFV infection compared with serum from patients without pneumonia, suggesting that serum cytokine dynamics are associated with prognosis in influenza-related pneumonia [35] . Therefore, the present research raises the possibility that reduced IL-6 production under Dectin-2 deficiency may affect suppression of the severity of pneumonia caused by harmful cytokine storms. This hypothesis may lead to the development of novel therapeutics targeting Dectin-2 in life-threatening viral pneumonias, such as ARDS, or the outbreak of a new IFV pandemic. Further detailed investigation may be necessary to clarify the function of Dectin-2 in the inflammatory responses, focused on humoral factors using a mouse model of IFV infection.
TLR7 expressed in intracellular endosomes of pDC recognizes single-stranded DNA, which activates signal-transducing adapter molecule interferon regulating factor (IRF) 7 and promotes type I IFN production [36] . However, it is not well understood how the CLR signaling pathway is involved in type I IFN secretion by pDCs. Regarding the relationship between CLRs and type I IFN, analysis of the IFV infection model using CARD9-deficient mice showed no significant difference in IFN-α production in the lungs between WT and CARD9 KO mice. Still, the pathological findings in the infected lungs were clearly attenuated in CARD9 KO mice [8] . In the present study, IFN-α production by pDCs was increased under Dectin-2 deficiency compared with control mice, whereas IL-12p40 concentration in the same supernatant was significantly decreased (Figure 4). Previous reports have shown that high levels of IFN-α/β secreted by CD8α+ DCs suppressed IL-12 production from CD11b+ DCs in murine cytomegalovirus infection [37] . The present data suggest a possible mechanism by which enhanced IFN-α production under Dectin-2 deficiency promotes antiviral responses, suppressing viral replication and inflammatory responses.
Finally, we summarize the involvement of CLRs in IFV infection based on previous reports and our findings (Figure 6). In addition to Clec5a described in the Introduction section, mannose receptor and macrophage galactose lectin (MGL) have been shown to function as co-receptors in intracellular viral entry via multiple polysaccharides on the surface of IFV particles [38] [39] [40] .
![]()
Figure 6. Summary of the involvement of transmembrane C-type lectin receptors (CLRs) in the interaction with surface polysaccharides modified on influenza virus (IFV). Dectin-2 senses mannose polysaccharide, which is abundant in hemagglutinin (HA), and promotes the production of proinflammatory cytokines such as interleukin (IL)-6 and IL-12 by conventional dendritic cells (DCs) through the activation of the intracellular signaling molecule spleen tyrosine kinase (Syk). In contrast, this receptor may suppress interferon (IFN)-α production by plasmacytoid DCs. Other CLRs support sialic acid-independent viral entry into the host cells and subsequent viral replication through the recognition of viral polysaccharides. However, the mechanism of intracellular signaling pathway triggered by ligand ligation is unknown. Clec5a has also been shown to enhance the inflammatory response in the lungs caused by IFV infection, but the glycan that binds to Clec5a has not yet been characterized clearly.
However, the intracellular signaling pathways initiated by these receptors are unknown, and how they contribute to the host inflammatory responses triggered by IFV is not clear. In the present study, more robust evidence was obtained regarding the mechanism by which Dectin-2 recognizes mannose polysaccharides in IFV-derived HA, leading to the initiation of host primary inflammation. In addition, it has been reported that different glycan structures on HA affect the function of the neutralizing antibody response against IFV. Although multiple polysaccharides are modified in HA in chicken egg-derived vaccines, the enhancement of an HA-specific antibody response, such as improved cross-reactivity and neutralization activity post infection with IFV, has been observed by monoglycosylation against various types of A/H1N1 subtype IFV [41] . The former report that the type and quantity of polysaccharides on HA affect vaccine efficacy suggests that Dectin-2 may be essential in the host defense mediated by mannose polysaccharides against IFV infection. Further analysis is necessary to elucidate the molecular dynamics of Dectin-2 in the pathogenesis of IFV infection and the HA-specific antibody response.
Acknowledgements
This work was supported by JSPS KAKENHI (grant numbers JP20K17461, JP22K08577), and by a research grant from the Takeda Science Foundation.
Supplement
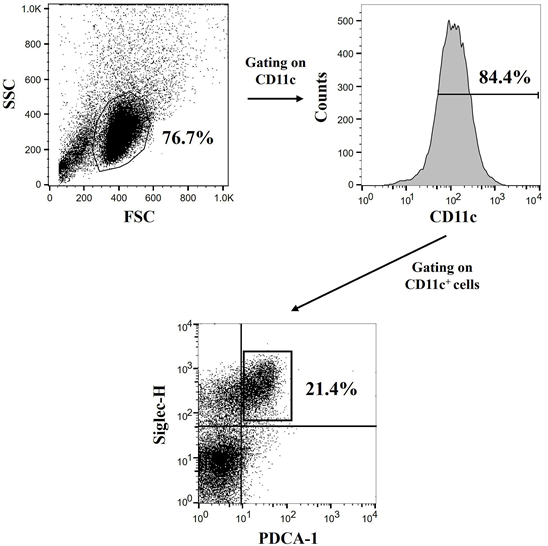
Supplemental Figure 1. Definition of bone marrow-derived plasmacytoid or convectional DCs. Bone marrow cells from wild-type mice were co-cultured with FMS-like tyrosine kinase 3 ligand (Flt-3L; 100 μg/mL) for 5 days and non-adherent cells were collected. The harvested cells were blocked with anti-mouse CD16/CD32 antibody and stained with fluorescein isothiocyanate (FITC)-labeled anti-mouse CD11c, phycoerythrin (PE)-labeled anti-mouse Siglec-H, and allo-phycocyanin (APC)-labeled anti-mouse PDCA-1. The CD11c+Siglec-H+PDCA-1+ cells were de-fined as plasmacytoid DCs and CD11c+Siglec-H−PDCA-1− cells as conventional DCs by flow-cytometric analysis.