Water Stress Effect on Biochemical Processes of Aus Rice Genotypes ()
1. Introduction
Water deficit is a major problem in growing rice, especially in the low rainfall seasons [1]. Biochemical processes were massively affected by drought and the activities of antioxidant enzymes and proline accumulation were associated with the dry mass production and consequently with the drought tolerance of the upland rice varieties [2]. It was also reported that water deficit is one of the major environmental threats to rice (Oryzasativa L.) cultivation and production [3]. Rice is more susceptible to drought than any other crop. It is estimated that the world needs to produce 40% more rice to feed the population by 2025 [4].
In agriculture, mild to severe drought has been one of the major production limiting factors. Drought reduces plant growth by affecting various physiological and biochemical processes, such as photosynthesis, respiration, translocation, ion uptake, carbohydrates and nutrient metabolism, and growth promoters [5]. Metabolic changes during drought affect the reduction of nutrients such as carbohydrates, nitrates, and potassium concentration decreases [6]. It is also suggested that the high relative water content could help the tolerant variety to perform physio-biochemical processes more efficiently under water stress treatments than the susceptible variety [7]. Progressive drought stress induced a considerable accumulation of proline in water stressed maize plants. Proline accumulation was the first response of plants exposed to water-deficit stress in order to reduce injury to cells [8]. In the case of rice, shoot accumulate proline in water stress conditions, and also ABA accumulates in water stress conditions [9].
Anthocyanin content was increased under water stress conditions. Leaf accumulates anthocyanins under drought conditions and the color increased as the intensity of water deficit conditions. Anthocyanin was water-soluble pigment found in all plant tissues [10]. Anthocyanins had been located in the root, shoot and leaves. Anthocyanins had been found in or just below the upper epidermis of leaves and often appear at specific developmental stages found that anthocyanins usually appear red in leaf cells, but depending on their chemical nature and concentration, the vacuolar pH, and interactions with other pigments, they can result in pink, purple, blue, orange, brown, and even black leaf colors [11] [12].
There is an urgent need to increase rice production to meet global demand [4]. Severe water stress may result in the arrest of photosynthesis, disturbance of metabolism and finally, the death of plants [5]. Hence, water stress management strategies need to be taken for better yield and improved varieties that are more resilient to abiotic stresses. Agricultural technology related to crop production has to be developed according to specific location. Considering the above-mentioned facts, the present research work was undertaken to achieve the following objectives to find out the responses of biochemical processes of aus rice genotypes under water deficit conditions.
2. Materials and Methods
2.1. Experimental Site
The experiment was conducted at the Plant Physiology Laboratory (central laboratory) and Agricultural research field of Agricultural Botany, Sher-e-Bangla Agricultural University, Dhaka-1207, Bangladesh under polythene shed controlling the intrusion of rainfall.
2.2. Experimental Period
The experiment was conducted during the period from March 2014 to July 2014
2.3. Genotypes and Treatments
Three BRRI materials as BRRI dhan55 (V1), BR6976-2B-15 (V2) and tolerant check Hashikalmi (V3) were collected from Genetic Resource and Seed Division, Bangladesh Rice Research Institute. Seven drought conditions were used as treatments that started from 20 days of seedling age.
T0 = Irrigated continuously throughout the experimental period (control).
T1 = Drought condition during 7 days, when the age of the seedling was 20 days.
T2 = Drought condition was applied for second 7 days, when the age of the seedling was 35 days.
T3 = Drought condition was applied for third 7 days, when the age of the plant was 55 days.
T4 = Drought condition was applied for fourth 7 days, when the age of the plant was 75 days.
T5 = Drought condition was applied for fifth 7 days, when the age of the plant was 95 days.
T6 = Drought condition was applied for sixth 7 days, when the age of the plant was 115 days.
2.4. Experimental Design, Replications
The experiment was designed as RCBD, three replications, three genotypes and seven water deficit treatments (63 pots) were used.
2.5. Seed Treatment and Sowing
Seeds of uniform size and shape of each genotype were treated with Bavistin 5 gm for 20 minutes. The solution was prepared by dissolving 5 g of Bavistin in 1/2 liter of water. Treated seeds were place in the Petridish with water (Plate 1). Pre-soaked sprouted seeds were sown on March, 2014 in earthen pots under the rain protected polyethylene shade.
2.6. Pot Preparation and Fertilizer Management
Earthen pots of 38 cm × 25 cm in size were used and filled up with 10 kg sandy loam soil. The soil of the experimental area was sandy and sandy loam (Plate 2). The soil of the pot was fertilized uniformly with 0.9, 0.8, 0.8 g urea, triple super phosphate and muriate of potash corresponding to 160-150-150 kg urea, triple super phosphate and muriate of potash per hectare, respectively [13]. Experimental duration was March to July, 2014. After seedling establishment, three uniform and healthy seedlings were allowed to grow per pot. The sprouted seeds were normally irrigated for ensuring normal growth. Water stress was imposed for seven days, when the age of the plant was 20, 35, 55, 75, 95 and 115 days.

Plate 1. Treated seeds were place in the Petridish with water.
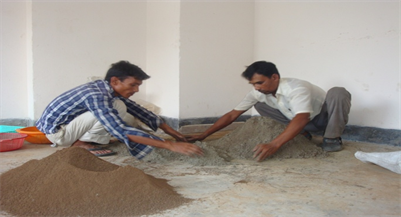
Plate 2. Soil preparation, filled up with 10 kg sandy loam soil and fertilized.
2.7. The Biochemical Parameters Determined
Plant growth under drought were influenced by altered photosynthesis, respiration, translocation, ion uptake, leaf proline accumulation, soluble sugar (carbohydrates), insoluble sugar (starch), nutrient metabolism, and hormones. In this study, leaf proline accumulation, soluble sugar (carbohydrates), insoluble sugar (starch), proline was determined in the Agricultural Botany laboratory under central laboratory of Sher-e-Bangla Agricultural University.
1) Leaf proline accumulation
At the end of the stress, the middle portion of flag leaf was collected for proline estimation following nin-hydrin method [14]. The protocol is based on the formation of red colored form zone by proline with ninhydrin in acidic medium, which is soluble in organic solvents like toluene.
Instruments, glassware and reagents
Test-tube, Test-tube stand, micro-pipettes (20 - 200 ml, 100 - 1000 ml and 5 ml), Whatman No. 1 filter paper, visible range spectrophotometer, centrifuge tube, centrifuge machine. Reagents were Glacial acetic acid (Analytical grade), Salfosalycylic acid (3%): Three gram of sulphosalycylic acid was dissolved in 100 ml of distilled water. Orthophoohoric acid (6 N): Required volume of orthophoric acid (38.1) were taken the volume were made to 100 ml, using distilled water to get 6 N orthophoohoric acid. Ninhydrin: Ninhydrin (1.25 g) were dissolved in a blend of 30 ml of glacial acetic acid 20 ml of 6N orthophoric acid.
Procedure
1) Plant tissue 0.5 g was homogenized in 5 ml of 3% sulphosalycylic acid using pre washed mortar and pestle.
2) The homogenate was filtered through Whatman No. 1 filter paper and collect filtrate for the estimation of proline content.
3) 2 ml of extract were taken in the test tube and add 2 ml of glacial acetic acid and 2 ml of ninhydrin reagent.
4) Heat reaction mixture in a boiling water bath at 100˚C for 1 hour. Brick red color was developed.
5) After cooling the reaction mixtures, 4 ml of toluene were added and then transferred to a separating funnel.
6.) After mixing, the chromospheres containing toluene were separated and absorbance was read at 520 nm in spectrometer against toluene blank.
7) Standard curve of proline was taking 5 to 100 µg·ml−1 concentration.
8) Free proline content was estimated by referring to a standard curve made from known concentrations of proline by taking following formula.
Preparation of proline standard curve
80 mg of pure proline was dissolved into 100 mL of distilled water to get 800 ppm proline stock solution for preparing proline standard curve. By diluting this solution, 50 ppm, 100 ppm, 200 ppm, 400 ppm and 800 ppm solution were prepared in 20 mL each. The absorbance was measured with the help of Spectrophotometer at 520 nm. By plotting the concentration of proline (ppm) in “X” axis and obtained absorbance reading in “Y” axis a standard curve was prepared. From the absorbance reading obtained from samples, their respective proline content was estimated in ppm by using proline standard curve and converted into micro gram per gram (µg/g) unit using the following formula:
2) Estimation of soluble sugar
Dried composite sample were grounded finely with mortar and pastel. 100 mg finely ground sample place in 15 ml centrifuge tube and 10 ml of 80% ethanol were added. The tubes were covered with aluminum and kept in a boiling water bath 80˚C - 85˚C for 30 minutes. Then the sample were centrifuged at 5000 rpm (by G-13 Centrifuge Machine, Model: NF 200, Turkey) for 10 minutes at room temperature) and decanted in to a 50 ml beaker. These extractions were repeated three or more 3 times. The alcohol extract was evaporated on a boiling water bath 80˚C - 85˚C until most of the alcohol were removed (the volume was reduced to about 3 ml.). The volume was made up to 25 ml with distilled water. 5 ml of this diluted sugar extract were taken in to 100 ml volumetric flask and should be made up the volume with distilled water. 5 ml diluted sugar take into Pyrex test tube which containing standard glucose (0, 0.01, 0.025, 0.05, 0.1, 0.2 and 0.3 mg/5ml) were put into ice bath. 10ml anthron reagents were added slowly in each test tube. Anthron reagents were prepared, 2 g anthrone were dissolved in one-liter concentrated sulfuric acid and stored in a refrigerator and a fresh solution. A Fresh solution was prepared every day. Reagent run down the side of the test tube and stirred slowly with a glass rod. Then the tubes were put in boiling water bath for exactly 7.5 minutes and cool down immediately in ice. Then the absorbance measured at 630 nm and sugar content was estimated using standard curve.
Calculation:The regression equation obtained from the standard curve is given below: Y = 0.3x – 0.14, Where, Y = absorbance at 630 nm, X = amount of glucose in mg
The amount of sugar in the extracts was obtained from the regression equation by putting the respective absorbance values of the extracts and taking into consideration the appropriate dilution factor.
3) Estimation of starch
The residue left in the centrifuge tube after collecting starch extract were dried in an oven at 80˚C and 2 ml distilled water were added to each centrifuge tube. The tubes were put in boiling water bath for 15 minutes and stirred occasionally. Then the tubes were allowed to cool and 2 ml 9.2 N HClO4 (perchloric acid) were added to each tube while were stirred occasionally or 15 minutes and made up 10 ml of distilled water. The supernatant was collected and add 2 m 4.6 N HClO4 were added to the residue and stirred for 15 minute and the solution made up 10 ml distilled water. Then suspension centrifuge and made up 50 with distilled water. 5 ml starch extract were transferred to 50 ml volumetric flask and made up the volume with distilled water. 5 ml diluted extract starch taken into separate 50 ml Pyrex test tubes which contains standard glucose (0.1, 0.25, 0.50, 0.75, 1 and 1.5 mg/5ml including 0.6 ml 0.46 N HCl04 solution for each tube) were put into ice bath and add 10 ml anthron reagent were added slowly, the reagent being allowed to run down the side of the test tubes. The solution was stirred slowly with a glass rod. Then the tubes were put in boiling water bath for exactly 7.5 minutes and cool down immediately in ice. Then the absorbance measured at 630 nm and sugar content was estimated using standard curve.
Calculation
The regression equation obtained from the standard curve is given below:
where, Y = absorbance at 630 nm.
X = amount of glucose in mg.
The amount of starch in the extracts was obtained from the regression equation by putting the respective absorbance values of the extracts and taking into consideration the appropriate dilution factor.
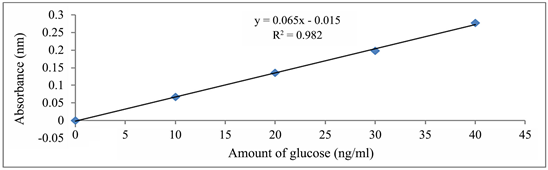
Calibration curve for the estimation of starch concentration
Extraction of anthocyanins
According to [15], anthocyanins were extracted from rice leaves. In the study, total anthocyanins were extracted from rice leaves using methanol and 1% HCL. Just after collection fresh sample were grounded finely with mortar and pastel. After grinding, samples were incubated overnight at room temperature in extraction buffer. 0.5 g finely ground sample place in 15 ml centrifuge tube and 60 ml of methanol were added with 1.6 ml HCL. Homogenates/ extracts were centrifuged at 16,000 g for 15 min and absorption of the extracts was determined at 530 and 657 nm by spectrometer. Then, anthocyanins were quantified according to the formula proposed by [15] as follows: QAnthocyanins = (A530 – 0.25 × A657) × M−1; QAnthocyanins: amount of anthocyanins, A530: absorption at 530 nm wavelength, A657: absorption at 530 nm wavelength, M: fresh weight (g) of the tissues, (60 ml MEOH and 1.6 ML 37% HCL).
Data analysis
The data were statistically analyzed following MSTAT-C software package and the mean differences were adjusted by Duncan’s Multiple Range Test (DMRT) at 5% level of significance [16].
3. Results and Discussions
The results with discussion of the experiments are presented in this chapter.
3.1. Proline Accumulation
Proline content (µg/g) of different rice genotypes under drought condition has been shown (Tables 1-3). Significant difference among the genotypes, the treatments and interaction effect for the character of proline content were found. In this study, in case of varietal affect the highest proline content found was 2.15 (µg/g) in BRRI dhan55 (V1) followed by 2.14 (µg/g) in Hashikalmi (V3) and the lowest proline content found was 2.13 in BR6976 2B-15. In case of interaction effect the highest proline content found was 2.26 (µg/g) in V1T0 and the lowest proline content found was 1.4 (µg/g) in V3T3 and in V2T6 under drought condition.
Due to drought condition (Plate 3) higher proline accumulation in BRRI dhan55 (V1) and comparatively lowest proline content was in BR6976 2B-15 (V2) which was significantly different among the genotypes. This result agrees with the result of [8] that proline accumulation was the first response of plants exposed to water-deficit stress in order to reduce injury to cells. Progressive drought stress induced a considerable accumulation of proline in water stressed maize plants. A similar finding was observed with the results of [17] that tolerant cultivar showed the highest accumulation of proline in bean plants. The highest accumulation of proline due to water stress (Plate 4 and Plate 5) was observed in genotype BB24 (382%) followed by BB43 (368%) and the lowest accumulation was in BB04 (163%). Genotype BARI bushbean-2 exhibited an intermediate behavior in proline accumulation. Accumulation of proline content under water stress indicates accumulated proline might act as a compatible solute regulating and reducing water loss from the plant cell during water deficit [18]. Decreasing of turgor pressure is the first reason for proline accumulation under drought stress condition. One of the physiological responses that plants use against drought is proline accumulation [19]. The proline accumulated in plants under water stress can protects the cell by balancing the osmotic potential of cytosol with that of vacuole and external environment [20]. The level of increase in the proline concentration in response to water stress varied between the rice varieties. Thus, the proline content (Plate 6) is a good indicator for screening drought tolerant varieties in water stress condition [21] and [22].
![]()
Table 1. Varietal effects of proline, soluble sugar and starch content genotypes under water stress condition.
Values followed by some letter(s) indicate significantly different from each other by DMRT at 5% level.
![]()
Table 2. Treatment effects of proline, soluble sugar and starch content genotypes under water stress condition.
Values followed by some letter(s) indicate significantly different from each other by DMRT at 5% level.
![]()
Table 3. Interaction effect of proline, sugar and starch of three rice genotypes under water stress condition.
Values followed by some letter(s) indicate significantly different from each other by DMRT at 5% level.

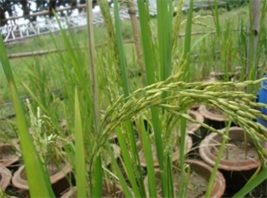
Plate 3. Different rice genotypes before drought treatment at reproductive stage.
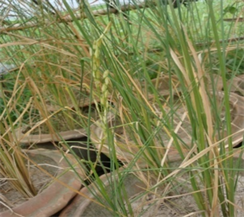
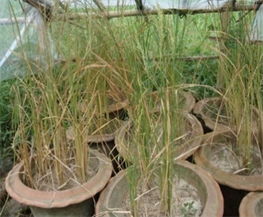
Plate 4. Different rice genotypes after water stress treatment at reproductive stage.
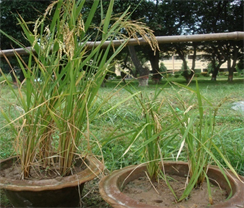
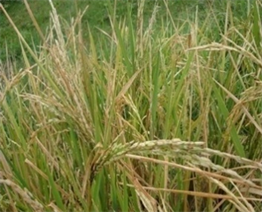
Plate 5. Effect of water stress of different rice genotypes at reproductive stage.
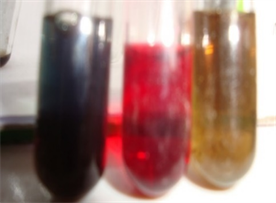
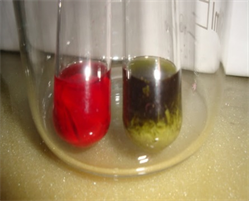
Plate 6. Leaf proline analysis of different rice genotypes under water stress conditions.
3.2. Soluble Sugar Content
Soluble sugar content of leaf of different rice genotypes under drought condition have been shown in the Tables 1-3. Significant difference among the genotypes, the treatments and interaction effect for soluble sugar content were found. In case of varietal effect, the highest sugar content found was 0.20 mg/g in BRRI dhan55 (V1) followed by 0.19 mg/g Hashikalmi and the lowest sugar content found was 0.18 mg/g in BR 6976-2B-15 (V2). In case of treatment effect, the highest varietal effect of proline, soluble sugar and starch content of three rice sugar content found was 0.199 mg/g in T0 and the lowest sugar content found was 0.13 mg/g in T5. In case of combination effect the highest sugar, content found was 0.200 mg/g in V2T0 and the lowest sugar content found was 0.112 mg/g in V2T2. Due to water stress conditions, soluble sugar content of leaf of different rice genotypes was found significantly much lower. BRRI dhan55 and tolerant check Hashikalmi which was less affected and the lowest soluble sugar content was found in BR 6976-2B-15 (V2). The soluble sugar content was much lower under water stress condition and this might be due to lower RWC, chlorophyll content, stomatal conductance and higher leaf rolling. Similar observations have been reported [15] who showed that under severe drought, growth was inhibited by high concentration of ABA and sugar, whereas low concentrations promote growth. ABA applications enhanced the percentage recovery of drought plants.
3.3. Starch Content
Starch content of different rice genotypes under drought condition have been shown in Tables 1-3. In case of varietal effect, the highest starch content found was 0.067 mg/g in Hasikalmi (V3) followed by 0.057 mg/g in BRRI dhan55 (V1) and the lowest starch content found was 0.054 mg/g in BR 6976-2B-15 (V2) which was significant difference among the genotypes. In case of treatment effect, the highest starch content was 0.063 mg/g in T6 and the lowest starch content was 0.054 mg/g in T0 which were significantly different among the treatment. In case of combination effect the highest starch, content found was 0.067 mg/g and the lowest starch content found was 0.053 mg/g which were not significantly different from each other. Due to water stress treatments starch content of different rice genotypes was significantly different among the genotypes. But the reduction was comparatively lower in BR 6976-2B-15 (V2) compared to other genotypes. Among the genotypes, in drought plant, the starch content was also found comparatively higher in BRRI dhan55 among the genotypes under water stress conditions, due to limitation of gas diffusion and reduced stomatal conductance, the production of carbohydrate is hampered. So, the rice genotypes that maintained higher carbohydrate before drought, they develop new leaves more quickly and accumulated greater biomass during recovery [23].
Anthocyanin content of leaf
Anthocyanin content (QAnthocyanins = (A530 – 0.25 × A657) × M−1) of different rice genotypes under drought condition (Plate 7) have been shown in Figure 1. Here the highest anthocyanin content was found in 0.464 in BRRI dhan55 (V1) followed by 0.402 in Hashikalmi (V3) and the lowest found was 0.305 in BR6976-2B-15 (V2). Anthocyanin content was increased under water stress condition. The lowest anthocyanin content was 0.0062 which was well water green color leaf.
In the study, anthocyanin content was increased under water stress condition. Leaf accumulates anthocyanins under drought conditions and the red color increased as the intensity of water deficit increased. Under drought condition BRRI dhan55 (V1) produced more anthocyanins followed by Hashikalmi (V3) to survive plant against stress conditions. This result has the similarity with the results who stated that anthocyanin were water soluble pigments found in all plant tissues due to stress condition [10]. Anthocyanins usually appear red color in
![]()
![]()
Plate 7. Leaf anthocyanin analysis of different rice genotypes under water stress conditions.
![]()
Figure 1. Anthocyanin content of three rice genotypes under water deficit conditions.
leaf cells due to stress condition, but depending on their chemical nature and concentration, the vacuolar pH and interactions with other pigments, they can result in red, pink, purple, blue, orange, brown, and even black leaf colors [24]. Anthocyanins had been located in the root, shoot, and leaves [11] [12]. Anthocyanins had been found in or just below the upper epidermis of leaves published the articles that coloration has assumed red foliage to be the outcome of the production of anthocyanins on plant [25].
Anthocyanins are polyphenol compounds a group of polyphenolic pigments found in nature, which contribute vivid colors to many fruits and vegetables and act as photo protective light screen, quenching excess photons and, thereby, mitigate photo inhibitory and photo-oxidative damage [26] and [27]. Anthocyanins neutralize reactive oxygen and nitrogen species compounds ascorbic acid and α-tocopherol do [28]. Anthocyanins are synthesized from the flavonoid biosynthetic pathway derived from the general phenylpropanoid pathway. Flavonoids play important roles in plants, such as pigmentation, plant reproduction by recruiting pollinators and seed dispersers and by stress protection. Anthocyanins scavenge free radicals and reducing the oxidative stress. Anthocyanins act as H-atom donator or as single electron transfer. Anthocyanin molecules will change their color depending upon the pH of their environment [29]. Anthocyanins are photo protective agents which shade and protect the photosynthetic apparatus by absorbing excess visible and UV light and scavenging free radicals [30].
Anthocyanins have a higher antioxidant activity than other flavonoids, due to their positively charged oxygen atom [31]. The antioxidant activity of anthocyanins depends on the degree of hydroxylation at the B-ring as well as the type and extent of acylation and glycosylation [32]. Hydroxylation at the B-ring enhances antioxidant capacity (−OH > −OCH3
−H), therefore the antioxidant capacity of anthocyanidins decreases in the order of Dp > Pt > Mv = Cy > Pn > Pg [33]. Furthermore, glycosylation reduces the free radical scavenging ability of anthocyanins compared to their aglycone forms, by decreasing their hydrogen-donating, metal-chelating and electron delocalizing abilities [34]. The more sugar units at C3 and C5 position, the lower the antioxidant activity is [32]. Finally, acylation of glycosyl moieties may partly circumvent the negative effect of glycosylation [35]. In summary, antioxidant activity increases with the number of hydroxyl groups in the B-ring and decreases with the number of glycosyl groups attached to the A and C ring. The latter effect is less severe when the glycosides are acylated.
The photo protective role of anthocyanins is seen in juvenile expanding leaves, because anthocyanin accumulation correlates with the development of photo stability [36]. Anthocyanins are thought to act as osmoregulatory under drought stress, because many drought-tolerant plant species contain anthocyanins [37]. In turn, plant tissues containing anthocyanins are usually rather resistant to drought [37]. Consistently, drought stress decreases chlorophyll concentrations [38] and [39]. Furthermore, soluble protein concentrations are reported to decrease under drought stress [38] and [39].
4. Conclusion
From the above results, the conclusion may be drawn as BRRI dhan55 and Hashikalmi showed better performance in all day’s water stresses. Under water stress conditions, proline was accumulated, and sugar and starch were decreased.
Acknowledgements
We thank anonymous reviewers for their kind review of the manuscript. This research was supported by Sher-e-Bangla Agricultural University Research System (SAURES) and HEQEP (Higher Education Quality Enhancement Project for providing financial support to conduct the research work.
Authors’ Contributions
This work was carried out in collaboration among all authors. Author HSJ conducted the research work. KUA designed and supervised the study and edited the manuscript. Author HSJ managed the literature searches and JKB collected genotypes from Genetic Resource and Seed Division, Bangladesh Rice Research Institute. All authors read and approved the final manuscript.