Mannich-Type Reaction of Aldimines with 2-Silyloxydienes Catalyzed by Ammonium Chloride ()
1. Introduction
Mannich-type reactions are widely recognized as a powerful method for constructing a variety of b-aminoketones [1] - [6]. However, Mannich-type reaction of imines with 2-silyloxydienes, which provides easy access to β-aminoketones having a terminal olefin, is still challenging because [4 + 2] type cycloadducts [7] - [15] or mixtures of Mannich-type products and cycloadducts [16] [17] [18] are obtained in most cases, as shown in Figure 1. Previously we first reported a highly effective Mannich-type reaction of imine with 2-silyloxydiene in the presence of zinc triflate and water [19] [20] [21] [22], which gave the corresponding β'-amino-α,β-enones as attractive skeletons for pharmaceutically useful compounds [23] [24] [25] [26]. Although many vinylogous Mannich-type reactions have been developed [27] - [36], only a few examples that describe the selective preparation of β'-amino-α,β-enones by the reaction of imine with 2-silyloxybutadiene have been reported so far. Thus, Kawęcki isolated the open-chain products from the aza-Diels-Alder reaction of sulfinimines with the
![]()
Figure 1. Reaction of imine with 2-silyloxydiene.
Rawal diene [37]. Pan et al. reported the addition of an α,β-unsaturated ketone-derived enolate to chiral N-phosphonyl imines [38], and Prasad et al. developed the reaction of chiral sulfinimines with silyloxydiene using TMSOTf [39]. In spite of these recent achievements, a more economical and environmentally benign synthetic methodology using green and sustainable catalysts has not been reported yet that offer alternatives to metal catalysts. Here we report the ammonium chloride-catalyzed Mannich-type reaction of imines with 2-silyloxybutadienes under mild conditions.
2. Results and Discussion
Initially, we examined the reaction of imine 1a, derived from benzaldehyde and o-anisidine, with 2-silyloxybutadiene 2a (Table 1). In contrast to the similar aza-Diels-Alder reaction of electron-rich Danishefsky’s diene, reported by Ding et al., which afforded the cyclic product in MeOH in the absence of any acids [40], the reaction of imine 1a with 2-silyloxybutadiene 2a in EtOH or MeOH without additives gave no product (Table 1, entry 1). This result suggested that
![]()
Table 1. Mannich-type reacton of imine (1a) with 2-silyloxydiene (2a)a.
aConditions: imine 1 (1 mmol), 2-silyloxydiene 2 (1.2 mmol), catalyst (0.1 mmol) in dry solvent (1 mL), r.t., 1 day. bIsolated yields.
using additive or catalyst was necessary to promote the reaction. We found that reaction with ammonium chloride (10 mol%) as a catalyst in EtOH gave the corresponding Mannich-type product 3a selectively in 95% isolated yield (entry 2). Interestingly, no trace of cycloadduct was detected by 500 MHz 1H NMR spectroscopy in the crude product. The previously reported reaction of imines having the N-benzyl group [19] did not give any products using ammonium chloride in EtOH, indicating that the reactivity of the imine is largely dependent on the N-protecting group. Finally, the attempt to perform the reaction using other additives and solvents was unsuccessful (Table 1, entries 3 - 7).
Having established the optimal reaction conditions for the Mannich-type reaction, we subsequently explored the scope of the reaction with respect to the imine substrates (Table 2). Imines 1b and 1c bearing an o- or p-tolyl group reacted to provide the corresponding products 3b (90% yield) and 3c (88% yield), respectively. Meanwhile, imines having an electron-donating or an electron-withdrawing group all reacted in a satisfactory way to provide the corresponding products 3d-3g in high yield. The reaction with 2-silyloxybutadiene 2b, derived from mesityl oxide, also proceeded to give 3h-3k in 87% - 97% yields. Further investigation of the reaction with 2-silyloxydiene 2c derived from acetylcyclohexene afforded the corresponding β'-amino-α,β-enones in 95% - 98% yields (Table 3).
To investigate the reaction mechanism, the reaction of 1a and 2a was quenched after 1 h and analyzed using 500 MHz 1H NMR spectroscopy. No cycloadduct was detected but a Mannich-type product and starting materials were observed. We suspect that the Mannich-type products are not formed via cyclization/ring-opening mechanism, as in the case of our previous reaction between N-benzyl-protected imine and 2-silyloxybutadiene using zinc triflate and water (Scheme 1) [19]. Additionally, further HCl work-up of the acyclic product 3a gave no cycloadducts but the β'-amino-α,β-enone was recovered. However, N-benzyl-protected acyclic products afforded piperidones upon reaction with HCl [19], indicating that the acyclic product 3a is stable under acidic conditions.
To demonstrate the synthetic utility of the Mannich-type products, we performed the preparation of a precursor of the prasugrel skeleton [41] [42], as
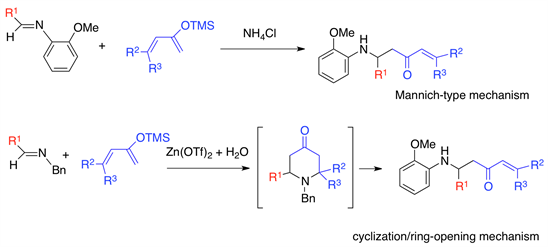
Scheme 1. Plausible mechanism.
![]()
Table 2. Scope of imines (1) and 2-silyloxydienes (2a)a.
aConditions: imine 1 (1 mmol), 2-silyloxydiene 2 (1.2 mmol), ammonium chloride (0.1 mmol) in dry EtOH (1 mL), r.t., 1 day. bIsolated yields.
shown in Scheme 2. Thus, the reaction of the imine (1h) derived from o-fluorobenzaldehyde with 2-silyloxybutadiene 2b proceeded smoothly to afford
![]()
Table 3. Reaction of imines (1) with 2-silyloxydiene (2c) derived from acetylcyclohexenea.
aConditions: imine 1 (1 mmol), 2-silyloxydiene 2c (1.2 mmol), ammonium chloride (0.1 mmol) in dry EtOH (1 mL), r.t., 1 day. bIsolated yields.
the corresponding acyclic product 3o, which was then cyclopropanated to give 4o in 65% yield.
3. Conclusion
In summary, a Mannich-type reaction of imine with 2-silyloxybutadiene that provides access to versatile β'-amino-α,β-enones has been developed under green conditions. We found that the use of ammonium chloride in EtOH is critical for the efficient outcome of the reaction, and this non-metal method is useful for various dienes and aldimines compared to reports so far [37] [38] [39]. According to our results, the reaction proceeds via Mannich-type mechanism, instead of through a cyclization/ring-opening process. Additionally, we prepared a precursor of the prasugrel skeleton by cyclopropanation of 3o. Further investigation of the applicability of this reaction and mechanistic elucidation is currently in progress.
4. Experimental
Typical Procedure for Mannich-Type reaction of imine 1 with 2-silyloxydiene 2
To a stirred solution of NH4Cl (0.006 g, 0.1 mmol), imine 1 (1 mmol) in dry ethanol (1 mL) was added 2-silyloxydiene 2 (1.2 mmol) at r.t.. The reaction mixture was stirred at r.t. for 24 h, the solvent was evaporated at reduced pressure. The crude product was purified by flash column chromatography (ethyl acetate: n-hexane = 1:6) to afford 3.
Data for 3a; colorless oil, 0.34 g, 95%. 1H NMR (CDCl3, 500 MHz) δ 3.17 (dd, 1H, J = 15.4 Hz, 5.7 Hz), 3.23 (dd, 1H, J = 15.4 Hz, 7.5 Hz), 3.85 (s, 3H), 4.97 (dd, 1H, J = 6.9 Hz, 6.9 Hz), 6.44 (d, 1H, J = 7.5 Hz), 6.45 - 6.76 (m, 4H), 7.22 - 7.25 (m, 1H), 7.32 - 7.49 (m, 10H); HRMS (EI) m/z [M]+ calcd. for C24H23NO2 357.1729, found: 357.1727.
Data for 3b; colorless oil. 0.30 g, 90%. 1H NMR (CDCl3, 300 MHz) δ 2.02 (s, 3H), 2.87 (dd, 1H, J = 15.4 Hz, 5.9 Hz), 2.95 (dd, 1H, J = 15.4 Hz, 6.9 Hz), 3.54 (s, 3H), 4.69 (dd, 1H, J = 6.6 Hz, 6.6 Hz), 6.23 (d, 1H, J = 6.9 Hz), 6.35 - 6.51 (m, 4H), 6.78 - 6.87 (m, 2H), 7.04 - 7.24 (m, 8H); HRMS (EI) m/z [M]+ calcd. for C25H25NO2 371.1885, found: 371.1894.
Data for 3c; colorless oil. 0.32 g, 88%. 1H NMR (CDCl3, 300MHz) δ 2.06 (s, 3H), 2.92 (dd, 1H, J = 15.4 Hz, 5.9 Hz), 2.96 (dd, 1H, J = 15.4 Hz, 7.0 Hz), 3.58 (s, 3H), 4.67 (dd, 1H, J = 6.3 Hz, 6.3 Hz), 6.23 (d, 1H, J = 7.7 Hz), 6.35 - 6.51 (m, 3H), 6.78 - 6.85 (m, 1H), 6.87 - 7.07 (m, 4H), 7.08 - 7.18 (m, 2H), 7.20 - 7.26 (m, 3H); HRMS (EI) m/z [M]+ calcd. for C25H25NO2 371.1885, found: 371.1902.
Data for 3d; colorless oil. 0.32 g, 85%. 1H NMR (CDCl3, 500 MHz) δ 3.12 (dd, 1H, J = 15.5 Hz, 5.7 Hz), 3.20 (dd, 1H, J = 15.5 Hz, 6.9 Hz), 3.74 (s, 3H), 3.83 (s, 3H), 4.91 (dd, 1H, J = 6.3 Hz, 6.3 Hz), 6.46 (d, 1H, J = 1.7 Hz), 6.61 - 6.63 (m, 4H), 6.81 - 6.85 (m, 2H), 7.25 - 7.37 (m, 5H), 7.42 - 7.47 (m, 3H); HRMS (EI) m/z [M]+ calcd. for C25H25NO3 387.1834, found: 387.1838.
Data for 3e; colorless oil. 0.36 g, 93%. 1H NMR (CDCl3, 300MHz) δ 3.12 (dd, 1H, J = 15.7 Hz, 5.5 Hz), 3.20 (dd, 1H, J =15.7 Hz, 5.5 Hz), 3.85 (s, 3H), 4.93 - 4.99 (m, 2H), 6.36 (d, 1H, J = 6.2 Hz), 6.61 - 6.76 (m, 4H), 7.26 - 7.53 (m, 10H); HRMS (EI) m/z [M]+ calcd. for C24H22NO2Cl 391.1339, found: 391.1328.
Data for 3f; colorless oil. 0.32 g, 81%. 1H NMR (CDCl3, 500 MHz) δ 3.02 (dd, 1H, J = 14.9 Hz, 8.6 Hz), 3.28 (d, 1H, J = 3.5 Hz), 3.71 (s, 3H), 5.28 (dd, 1H, J = 9.1 Hz, 3.4 Hz), 6.21 (dd, 1H, J = 6.2 Hz, 1.8 Hz), 6.59 - 6.74 (m, 4H), 7.17 - 7.59 (m, 10H); HRMS (EI) m/z [M]+ calcd. for C24H22NO2Cl 391.1339, found: 391.1335.
Data for 3g; colorless oil. 0.33 g, 87%. 1H NMR (CDCl3, 500 MHz) δ 3.13 (dd, 1H, J = 15.5 Hz, 5.7 Hz), 3.20 (dd, 1H, J = 15.5 Hz, 5.7 Hz), 3.83 (s, 3H), 4.93 (dd, 1H, J = 6.3 Hz, 6.3 Hz), 6.35 - 6.39 (m, 1H), 6.59 - 6.80 (m, 4H), 6.95 - 6.99 (m, 2H), 7.18 - 7.25 (m, 3H), 7.33 - 7.38 (m, 3H), 7.45 - 7.53 (m, 2H); HRMS (EI) m/z [M]+ calcd. for C24H22NO2 375.1634, found: 375.1628.
Data for 3h; colorless oil. 0.29 g, 95%. 1H NMR (CDCl3, 300 MHz) δ 1.72 (s, 3H), 2.00 (s, 3H), 2.76 (dd, 1H, J = 16.9 Hz, 5.9 Hz), 2.82 (dd, 1H, J = 16.9 Hz, 5.9 Hz), 3.81 (s, 3H), 4.77 (dd, 1H, J = 6.6 Hz, 6.6 Hz), 5.91 (brs, 1H), 6.30 - 6.35 (m, 1H), 6.45 - 6.55 (m, 1H), 6.58 - 6.64 (m, 2H), 7.06 - 7.15 (m, 1H), 7.17 - 7.20 (m, 2H), 7.26 - 7.28 (m, 2H); HRMS (EI) m/z [M]+ calcd. for C20H23NO2 309.1729, found: 309.1722.
Data for 3i; colorless oil. 0.31 g, 97%. 1H NMR (CDCl3, 500 MHz) δ 1.85 (s, 3H), 2.11 (s, 3H), 2.30 (s, 3H), 2.90 (dd, 1H, J = 13.2 Hz, 5.7 Hz), 2.93 (dd, 1H, J = 13.2 Hz, 5.7 Hz), 3.85 (s, 3H), 4.85 (dd, 1H, J = 6.9 Hz, 6.9 Hz), 6.04 (brs, 1H), 6.44 - 6.50 (m, 1H), 6.58 - 6.52 (m, 1H), 6.55 - 6.73 (m, 2H), 7.10 - 7.13 (m, 2H), 7.20 - 7.28 (m, 2H); HRMS (EI) m/z [M]+ calcd. for C21H24NO2 323.1885, found: 323.1894.
Data for 3j; colorless oil. 0.31 g, 92%. 1H NMR (CDCl3, 300 MHz) δ 1.80 (s, 3H), 2.08 (s, 3H), 2.80 (dd, 1H, J = 15.4 Hz, 6.2 Hz), 2.90 (dd, 1H, J = 15.4 Hz, 6.2 Hz), 3.70 (s, 3H), 3.80 (s, 3H), 4.81 (dd, 1H, J = 6.6 Hz, 6.6 Hz), 5.95 - 6.00 (m, 1H), 6.34 - 6.44 (m, 1H), 6.52 - 6.58 (m, 1H), 6.67 - 6.72 (m, 2H), 6.79 - 6.84 (m, 2H), 7.24 - 7.28 (m, 2H); HRMS (EI) m/z [M]+ calcd. for C21H25NO3 339.1834, found: 339.1836.
Data for 3k; colorless oil. 0.29 g, 89%. 1H NMR (CDCl3, 300 MHz) δ 1.85 (s, 3H), 2.10 (s, 3H), 2.88 (dd, 1H, J = 15.4 Hz, 5.9 Hz), 2.92 (dd, 1H, J = 15.4 Hz, 5.9 Hz), 3.11 (s, 3H), 4.84 (dd, 1H, J = 6.6 Hz, 6.6 Hz), 5.99 - 6.01 (m, 1H), 6.36 (d, 1H, J = 7.7 Hz), 6.59 - 6.76 (m, 3H), 6.95 - 7.01 (m, 2H), 7.32 - 7.37 (m, 2H); HRMS (EI) m/z [M]+ calcd. for C20H22NO2F 327.1635, found: 327.1628.
Data for 3l; colorless oil. 0.33 g, 98%. 1H NMR (CDCl3, 300 MHz) δ 1.50 - 1.65 (m, 4H), 2.15 - 2.28 (m, 4H), 3.12 (d, 2H, J = 6.6 Hz), 3.85 (s, 3H), 4.82 - 4.90 (m, 1H), 4.92 - 5.05 (m, 1H), 6.37 (dd, 1H, J = 5.9 Hz, 1.8 Hz), 6.57 - 6.84 (m, 4H), 7.18 - 7.39 (m, 5H); HRMS (EI) m/z [M]+ calcd. for C22H25NO2 335.1885, found: 335.1876.
Data for 3m; colorless oil. 0.27 g, 78%. 1H NMR (CDCl3, 300 MHz) δ 1.50 - 1.65 (m, 4H), 2.15 - 2.25 (m, 4H), 2.30 (s, 3H), 3.11 (d, 2H, J = 6.6 Hz), 3.85 (s, 3H), 4.82 (dd, 1H, J = 6.6 Hz, 6.6 Hz), 6.40 (d, 1H, J = 6.2 Hz), 6.57 - 6.75 (m, 3H), 6.83 - 6.86 (m, 1H), 7.10 (d, 2H, J = 8.0 Hz), 7.27 (d, 2H, J = 8.4 Hz); HRMS (EI) m/z [M]+ calcd. for C23H27NO2 349.2042, found: 349.2044.
Data for 3n; colorless oil. 0.34 g, 95%. 1H NMR (CDCl3, 300 MHz) δ 1.56 - 1.59 (m, 4H), 2.17 - 2.21 (m, 4H), 3.11 (dd, 2H, J = 5.9 Hz, 1.8 Hz), 3.86 (s, 3H), 4.80 - 4.87 (m, 1H), 4.96 (brs, 1H), 6.33 (dd, 1H, J = 7.7 Hz, 1.5 Hz), 6.59 - 6.77 (m, 3H), 6.82 - 6.87 (m, 1H), 6.93 - 7.02 (m, 2H), 7.33 - 7.37 (m, 2H); HRMS (EI) m/z [M]+ calcd. for C22H24NO2F 353.1791, found: 353.1797.
Data for 3o; colorless oil. 0.24 g, 97%. 1H NMR (CDCl3, 500 MHz) δ 1.85 (s, 3H), 2.09 (s, 3H), 2.92 (dd, 1H, J = 15.4 Hz, 7.7 Hz), 2.97 (dd, 1H, J = 15.4 Hz, 7.7 Hz), 3.86 (s, 3H), 5.14 - 5.18 (m, 1H), 6.06 - 6.07 (m, 1H), 6.40 - 6.45 (m, 1H), 6.58 - 6.65 (m, 1H), 6.68 - 6.80 (m, 2H), 7.00 - 7.08 (m, 2H), 7.17 - 7.25 (m, 1H), 7.35 - 7.37 (m, 1H); HRMS (EI) m/z [M]+ calcd. for C20H22NO2F 327.1635, found: 327.1637.
Cyclopropanation of 3o using trimethyloxosulfonium iodide
To a stirred solution of 3o (0.243 g, 0.74 mmol) in DMSO (1 mL) was added trimethyloxosulfonium iodide (0.22 g, 1.0 mmol) and NaH (0.024 g, 1.0 mmol) at r.t. The mixture was stirred for 1 day and quenched with ice-water (20 mL). The mixture was extracted with ether, washed twice with water, and the organic layers were dried by Na2SO4. The solvent was removed at reduced pressure to give the product 4o as a white solid (0.21g, 65%).
Data for 4o; 1H NMR(CDCl3, 500MHz) δ 0.71 - 0.72 (m, 1H), 0.86 (s, 3H), 1.06 (s, 3H), 1.15 - 1.16 (m, 1H), 1.75 - 1.80 (m, 1H), 3.06 (dd, 1H, J = 16.1 Hz, 5.7 Hz), 3.09 (dd, 1H, J = 16.1 Hz, 5.7 Hz), 3.77 (s, 3H), 5.08 (brs, 1H), 5.16 (dd, 1H, J = 6.3 Hz, 6.3 Hz), 6.40 - 6.42 (m, 1H), 6.60 - 6.65 (m, 1H), 6.71 - 6.74 (m, 2H), 7.01 - 7.05 (m, 2H), 7.17 - 7.25 (m, 1H), 7.36 - 7.37 (m, 1H); HRMS (EI) m/z [M]+ calcd. for C21H24NO2F 341.1791, found: 341.1796.
Acknowledgements
We acknowledge JEOL Ltd. and Nihon Waters K. K. for measurement of HRMS.