1. Introduction
Proteases constitute one of the most important groups of industrial enzymes, and account for at least 60% of all global enzyme sales [1] . They have diverse applications in a wide variety of industries such as detergent, food, pharmaceutical, leather, peptide synthesis, and for the recovery of silver from used X-ray films [2] [3] . Recent advances in biotechnology have also demonstrated the capacity of proteases to produce fish protein hydrolysates [4] [5] . The detergent industry has now emerged as a major consumer of several hydrolytic enzymes acting at alkaline pH. Detergent proteases account for at least a quarter of all protease sales throughout the world [1] [6] [7] . They are primarily used as detergent additives since they are biodegradable and since they increase performance/cost ratios [1] [3] . Moreover, although the enzymes selected for detergent composition have been subtilisins, they are not the ideal enzymes for detergents due to their low thermal stability and also because of their short shelf life [7] . Thus, it is relevant to search for proteases from new sources presenting high thermal stability, alkaline activity and more compatible with washing systems [8] [9] . These properties have already been observed in trypsin-like enzymes.
Shrimp by-products have been identified as an animal protein source of a great potential and also as an important source of chitin and asthaxanthin [10] . Only 65% of the shrimp is edible. The remainder is discarded as inedible waste (cephalothorax and exoskeleton).
Over the years, techniques have been developed for the recovery and exploitation of these by products in valuable biopolymers such as chitin and chitosan [11] [12] . These biomolecules are widely used in the food industry, pharmacy, textiles, chemical industries, etc. [13] . Conventionally, to extract chitin from crustacean shells, chemical processing for demineralization and deproteinization has been applied by treatment with strong acids and bases to remove calcium carbonate and proteins, respectively. However, the use of these chemicals may destroy chitin. To overcome the hazards from chemical treatments, alternative methods in the use of microorganisms [14] [15] or proteolytic enzymes [16] [17] for the deproteinization of crustacean wastes have been reported.
The present paper describes some biochemical characterization of the alkaline crude enzyme preparation from A. arilaitensis as well as its compatibility with commercial laundry detergents, oxidants, surfactant agents and organic solvents and its application in the deproteinization of shrimp wastes.
2. Materials and Methods
2.1. Arthrobacter arilaitensis Re117
Arthrobacter arilaitensis is one of the major bacterial species found at the surface of cheeses, especially in smear-ripened cheeses, where it contributes to the typical colour, flavour and texture properties of the final pro- duct. In model cheese experiments, the addition of small amounts of iron strongly stimulates the growth of A. arilaitensis, indicating that cheese is a highly iron-restricted medium. We suggest that there is a strong selective pressure at the surface of cheese for strains with efficient iron acquisition and salt-tolerance systems together with the abilities to catabolize substrates such as lactic acid, lipids and amino acids.
2.2. Culture Conditions
A. arilaitensis strain Re117 was previously isolated from the surface of a Reblochon cheese. This strain is the type strain of the species, and was deposited in the CIP public strain collection (Collection of Institut Pasteur) as strain CIP 108037. It was routinely grown at 25˚C in brain heart infusion broth (BHI, Biokar Diagnostics, Beauvais, France) under aerobic conditions (rotary shaker at 150 rpm, volume of broth equivalent to 20% of the volume of the conical flask).
The growth of the microorganism was estimated by the determination of colony-forming units (CFU/ml). All experiments were carried out in duplicate and repeated at least twice.
2.3. Enzyme Production
The initial medium M1 used for the production of proteases by A. arilaitensis Re117 consists of (g/l) Mirabilis Jalapa Tuber Powder (MJTP) 10.0, ammonium sulfate 2.0, CaCl2 0.5 and MgSO4・7H2O 0.5. Cultures were performed on a rotatory shaker (150 rpm) for 24 h at 25˚C in 250-ml conical flasks with a working volume of 25 ml. Media were autoclaved at 121˚C for 20 min. The growth of the microorganism was estimated by total plate count on nutrient agar. The culture medium was centrifuged at 12,000 × g for 15 min at 4˚C, and the cell-free supernatant was used for the estimation of proteolytic activity.
2.4. Time Course of Protease Production by A. arilaitensis Re117
To study the relation between protease production and the growth profile of the bacterium, 100 ml of the optimized and unoptimized production media (MCD) was inoculated in 1-L flasks, and the growth was measured at regular intervals by viable count (spread plate method) determination. The protease production at different time intervals was determined using the standard protease assay.
2.5. Polyacrylamide Gel Electrophoresis
Sodium dodecyl sulphate-polyacrylamide gel electrophoresis (SDS-PAGE) was carried out as described by Laemmli 1970 [18] , using 5% (w/v) stacking and 15% (w/v) separating gels. Samples were prepared by mixing the crude enzyme extract at 1:5 (v/v) ratio with the SDS-PAGE sample buffer (10 mM Tris-HCl (pH 8.0), 2.5% SDS, 10% glycerol, 5% β-mercaptoethanol and 0.002% bromophenol blue). The samples were heated at 100 for 5 min before loading in the gel. After electrophoresis, the gel was stained with 0.25% Coomassie Brilliant Blue R-250 in 45% ethanol-10% acetic acid and destained with 5% ethanol-7.5% acetic acid.
2.6. Detection of Protease Activity by Zymography
Zymography was performed in conjunction with SDS-PAGE according to the method described by Garcia- Carreno et al., [19] with slight modification. The sample was not heated before electrophoresis. After electrophoresis, the gel was submerged in 100 mM glycine-NaOH pH 9.0 buffer containing 2.5% (v/v) Triton X-100 for 30 min at 4 with agitation. The gel was then washed with 100 mM glycine-NaOH pH 9.0 buffer, to remove Triton X-100, and incubated, for 30 min at 50˚C, in 100 mM glycine-NaOH pH 9.0 buffer containing 1% (w/v) casein. Finally, the gel was stained with Coomassie Brilliant Blue R-250. The development of the clear zone on the blue background indicated the presence of protease activity.
2.7. Protease Activity Assay
Protease activity in the crude alkaline enzyme crude was measured by the method of Kembhavi et al. (1993) [20] using casein as a substrate. A 0.5 ml aliquot of the crude enzyme, suitably diluted, was mixed with 0.5 ml of buffer A containing 1% substrate (w/v), and incubated for 15 min at 50˚C.
The reaction was stopped by the addition of 0.5 ml of 20% (w/v) trichloroacetic acid. The mixture was allowed to stand at room temperature for 15 min and then centrifuged at 10,000 × g for 15 min to remove the precipitate. The acid-soluble material was estimated spectrophotometrically at 280 nm. A standard curve was generated using solutions of 0 - 50 mg/l tyrosine. One unit of protease activity was defined as the amount of enzyme required to liberate 1 μg of tyrosine per min under the experimental conditions used. Values are the means of three independent experiments.
2.8. Properties of the Enzyme
2.8.1. Effect of pH on Activity and Stability
The optimum pH of the crude protease was studied over a pH range of 5.0 - 12.0 using casein as a substrate at 50˚C.
For the measurement of pH stability, the crude enzyme preparation was incubated for 1 h at 30˚C in different buffers, and then the residual proteolytic activity was determined under standard assay conditions. The following buffer systems were used: 100 mM acetate buffer, pH 5.0 - 6.0; 100 mM Tris-HCl buffer, pH 7.0 - 8.0; 100 mM glycine-NaOH buffer, pH 9.0 - 11.0; 100 mM Na2HPO4-NaOH buffer pH 12.0.
2.8.2. Effect of Temperature on Activity and Stability
To investigate the effect of temperature, the activity was tested using casein as a substrate at the temperature range of 20˚C to 70˚C in 100 mM glycine-NaOH buffer, pH 9.0.
Thermal stability was examined by incubating the enzyme preparation for 60 min at different temperatures from 30˚C to 70˚C. Aliquots were withdrawn at desired time intervals to test the remaining activity at pH 9.0˚C and 50˚C. The non heated crude enzyme was taken as 100%.
2.8.3. Effects of Metal Ions on the Alkaline Proteolytic Activity
The influence of various metal ions at a concentration of 5 mM, on enzyme activity was investigated by adding the monovalent (Na+ or K+) or divalent (Mg2+, Hg2+, Ca2+, Zn2+, Cu2+, Ba2+ or Mn2+) metal ions to the reaction mixture.
The activity of the crude enzyme without any metallic ions was considered as 100%.
2.8.4. Effect of NaCl Concentration
Enzyme activity was assayed in the presence of NaCl at various concentrations (0% - 15% (w/v)). The relative enzyme activity was determined at 50˚C for 15 min, using casein as a substrate.
2.8.5. Effects of Enzyme Inhibitors on Protease Activity
The effects of enzyme inhibitors (5 mM), on protease activity were studied using phenylmethylsulfonyl fluoride (PMSF), β-mercaptoethanol, pepstatin A and ethylene-diaminetetraacetic acid (EDTA). The alkaline crude enzyme extract was preincubated with each inhibitor for 30 min at 25˚C, and then the remaining protease activity was tested using casein as a substrate. The activity of the enzyme assayed in the absence of inhibitors was taken as control.
2.8.6. Effects of Surfactants and Oxidizing Agents
The suitability of the crude protease as a detergent additive was determined by testing its stability towards some surfactants (SDS, Triton X-100, Tween 80) and oxidizing agents (sodium perborate). The enzyme preparation was incubated with different concentrations of additives for 1 h at 30˚C, and then the residual proteolytic activities were measured under standard assay conditions. The activity of the enzyme preparation, incubated under similar conditions without any additives, was taken as 100%.
2.9. Detergent Compatibility
The compatibility of the crude alkaline protease extract with liquid laundry detergents was studied using commercially available detergents: Dixan (Henkel-Spain), Nadhif (Henkel-Alki-Tunisia), Lav+ (STID-Tunisia), Carrefour (U.E/Geproduceerd-France) and Tex’til (U.E/Vervaardigd-Belgium). Commercial detergents were diluted 100-fold in tap water to simulate wash solution. The endogenous enzymes contained in these detergents were inactivated by heating the diluted detergents for 1 h at 65˚C prior to the addition of the enzyme preparation. The crude protease was incubated with different detergents for 1 h at 30˚C and 40˚C, and then the remaining activities were determined under the standard assay conditions.
The enzyme activity of a control, without detergent, incubated under the similar conditions, was taken as 100%.
2.10. Organic Solvent Stability Assay
The organic solvent stability of the enzyme was studied by incubating the crude enzyme with various organic solvents (50%; v/v; methanol, N,N-dimethylformamide (DMF), dimethylsulfoxide (DMSO), diethyl ether, hexane, acetone, and isopropanol) at 30˚C with shaking (150 rpm) for 30 days. Aliquots were withdrawn at desired time intervals to test the remaining protease activity. Crude enzyme diluted using buffer was considered as control [17] .
2.11. Shrimp Waste Deproteinization
Shrimp wastes (50%, w/v) were minced and cooked at 100˚C for 20 min to inactivate endogenous enzymes. The cooked sample was then homogenized in a Moulinex® blender for 2 min. The pH of the mixture was adjusted to 9.0, then, the shrimp wastes proteins were digested with the Re117 crude enzyme. After 3 hours incubation at 50˚C, the reaction was stopped by heating the solution during 20 min at 90˚C. The shrimp wastes protein hydrolysate was then centrifuged 20 min at 5000 × g to separate insoluble and soluble fractions. The solid phase was washed with distilled water and dried for 1 h at 60˚C.
Deproteinization (DP) was expressed as percentages and computed by the following equation as described by Rao et al. [21] .
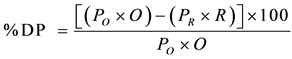
where PO and PR are protein concentrations (%) before and after hydrolysis, while O and R represent the mass (g) of original sample and hydrolyzed residue in dry weight basis, respectively.
2.12. Statistical Analyses
Statistical analyses were performed with Statgraphics ver.5.1, professional edition (Manugistics Corp., USA) using ANOVA analysis. Differences were considered significant at P < 0.05. All tests were carried out in triplicate.
3. Results and Discussion
3.1. Protease Production
In this study, protease production by A. arilaitensis strain was first tested in M1 medium containing different carbon sources at a concentration of 10 g/l.
A. arilaitensis strain exhibited a higher production level of protease in culture media containing SHVF (181 U/ml) as carbon source followed by chicken feather meal (96 U/ml) and SWP (91 U/ml). On the contrary, protease production between 27 and 2.18 U/ml was obtained with the other carbon sources tested (casein, hulled grain of wheat, MJTP, glucose, starch) (Figure 1(a)). Since the SHVF was a better carbon source, the effect of its concentration on protease production was studied. As shown in Figure 1(b), the highest protease production (495 U/ml) was achieved at 45 g/l.
The effect of various organic and inorganic nitrogen sources, at a concentration of 2 g/l, was examined in M1 medium containing 45 g/l of SHVF (Figure 2(a)). The various organic and inorganic nitrogen sources were tested and the maximum enzyme activity was obtained with pastone. A 3-fold higher production of protease by A. arilaitensis strain (1213 U/ml) was achieved when pastone was used at a concentration of 2 g/l.
Since pastone was the best nitrogen source for protease synthesis by A. arilaitensis, the effect of its concen- tration on the enzyme production was studied, and the maximum occurred at 5 g/l (2072 U/ml) (Figure 2(b)).
(a)
(b)
Figure 1. Effect of different carbon sources (a) and different concentrations of SHVF (b) on protease production by A. arilaitensis Re117.
(a)
(b)
Figure 2. Effect of different nitogen sources (a) and different concentrations of pastone (b) on protease production by A. arilaitensis Re117.
3.2. Time Course of Protease Production and Cell Growth
The time course of protease activity and the growth of A. arilaitensis Re117 for the Chemical defined medium (MCD) and at different temperatures are shown in Figure 3. These results show that the secretion of the proteases was associated with the cell growth. Protease activity increased during the exponential growth phase and reached activity of 61 U/ml in 24 h. The production of proteases increased slightly at the beginning of the stationary phase.
The maximum activity was obtained at 24˚C after 24 h and decreased gradually while increasing the temperature. At temperature 30˚C and 37˚C, the proteolytic activities reached 49 and 30 U/ml, respectively.
The obtained results indicate that MCD medium is an excellent medium for growth and not for protease production by A. arilaitensis. Figure 4 shows that the maximum protease activity reached 1330 U/ml within 24 h of optimum medium containing SHVF as a source of carbone. Since SHVF is cheaply obtained, its use as a carbon source instead of commercial substrates may reduce considerably the cost of enzyme production and could promote new industrial applications.
3.3. Sodium Dodecyl Sulphate-Polyacrylamide Gel Electrophoresis (SDS-PAGE) and Zymography of Crude Alkaline Protease
In order to estimate the number of proteases in the crude enzyme extract, sample was separated by SDS-PAGE, and then proteolytic activity was revealed by casein zymogram activity staining. As can be observed in Figure 5, the crude enzyme extract showed only one clear band of protease activity with different molecular weights. This result suggests that at least one proteinase was present in A. arilaitensis.
3.4. Effect of pH on Protease Activity and Stability
The relative activity values at various pHs from 3.0 to 12.0 are shown in Figure 6(a). The enzyme preparation was highly active between pH 6.0 and 11.0 with an optimum at pH 9.0 when incubated for 15 min at 50˚C. The relative activities at pH 8.0, 9.0 and 10.0 were about 98%, 100% and 87%, respectively. This characteristic is a relevant aspect that enables its use in liquid detergent formulations, as the pH of laundry detergents is commonly alkaline [22] . The optimum pH for A. arilaitensis proteases was similar to that reported by Manni et al. (2010) [17] for proteases from Bacillus cereus SV1.
The pH stability profile, reported in Figure 6(b), shows that the A. arilaitensis enzyme preparation is highly stable at a pH range between 8.0 and 9.0, maintaining 100% of its original activity after 1 h incubation at 4˚C.
(a)
(b)
Figure 3. Time course of growth (a) and protease production (b) of A. arilaitensis Re117 in the chemical defined medium at different temperature.
![]()
Figure 4. Time course of protease production of A. arilaitensis Re117 in the optimized medium.
![]()
Figure 5. SDS-PAGE (a) and zymogram (b) of the crude alkaline metalloprotease from A. arilaitensis Re117.
(a)
(b)
Figure 6. pH profile (a) and pH stability (b) of the crude alkaline protease from A. arilaitensis Re117. Protease activity was assayed in the pH range of 5.0 to 12.0 at 50˚C. The maximum activity obtained at pH 9.0 was considered as 100% activity. The pH stability was determined by incubating the enzyme in different buffers for 60 min at 30˚C, and the residual enzyme activity was determined at pH 9.0 and 50˚C using casein as a substrate. The activity of the enzyme before incubation was taken as 100%. Buffer solutions used for pH activity and stability are presented in “Material and Methods” section.
The residual activities at pH 10.0 and 11.0 were about 70% and 55%, respectively. These results suggest that the A. arilaitensis could be a potential source of proteases for certain industrial applications that require high alkaline conditions.
3.5. Effect of Temperature on Protease Activity and Stability
The effect of temperature on protease activity was determined by assaying enzyme activity at different temperatures (Figure 7(a)). The crude enzyme from A. arilaitensis was active at temperatures from 30˚C to 70˚C with an optimum between 40˚C and 60˚C. The relative activities at 40˚C and 60˚C were about 71% and 63%, respectively. However, an appreciable decrease in enzyme activity was observed above 60˚C, due to thermal denaturation. At 70˚C, the relative activity was only 40% of that of 60˚C. The optimum temperature for A. arilaitensis proteases was similar to that of Pseudomonas aeruginosa A2 protease [16] .
The thermal stability of A. arilaitensis protease is depicted in Figure 7(b). The residual activities at 40˚C, 50˚C and 60˚C were about 16%, 7% and 3%, respectively. Moreover, about 100% of the maximum activity was retained after incubation for 60 min at 30˚C.
3.6. Effects of Metal Ions on Protease Activity
Table 1 displays the effects of various metal ions at a concentration of 5 mM on the activity of crude enzyme from A. arilaitensis. The enzyme preparation proved completely sensitive to ion Hg2+. It is known that Hg2+ is among ions that act on sulfhydryl residues in proteins [23] . The inhibition caused by this ion suggests the relevance of sulfhydryl residues for the catalytic action of most proteases [24] . The effects of Cu2+ and Mn2+ (respectively, 71% and 78%) proved to be similar to those recorded for L. mormyrus enzyme (respectively, 82% and 69%) [25] . However, K+, Na+, Zn2+, Ca2+ and Mg2+ showed no influence on proteolytic activity. A similar result was observed by El-Hadj Ali et al. (2011) [25] where L. mormyrus crude enzyme retained 100% of its activity in the presence of K+, Na+ and Mg2+ when incubated under the same conditions.
3.7. Effect of NaCl Concentration
The effect of NaCl concentration on the activity of A. arilaitensis crude enzyme was studied at pH 9.0 and 50˚C by the addition of NaCl to the reaction mixture. As reported in Figure 8, a continuous decrease in proteolytic activity was observed with increasing NaCl concentration. The relative activity at 6% NaCl was approximately 5%. The decrease in activity could be explained by the precipitation of the enzyme caused by the “salting out” [26] . After 6% NaCl, the proteolytic activity was completly inhibited.
(a)
(b)
Figure 7. Temperature profile (a) and thermal stability (b) of the alkaline crude enzyme from the A. arilaitensis Re117. Enzyme activity was assayed at different temperatures ranging from 30˚C to 70˚C at pH 9.0, using casein as a substrate. The activity of the enzyme at 50˚C was taken as 100%. For thermal stability, the enzyme was incubated at different temperatures for 60 min. The residual enzyme activity was assayed at pH 9.0 and 50˚C. The non-heated enzyme was considered as control (100%).
![]()
Figure 8. Effect of NaCl concentration on the activity of the crude enzyme from A. arilaitensis Re117.
3.8. Effects of Enzyme Inhibitors on Protease Activity
The effects of various enzyme inhibitors, such as chelating agents and group-specific reagents, on enzyme activity were studied and reported in Table 1. EDTA, a metalloprotease inhibitor, completly affected the proteolytic activity of the crude enzyme. This indicated the importance of some metal ions in enzyme stabilization. On the other hand, β-mercaptoethanol, PMSF and DTNB were without influence on the enzyme activity.
3.9. Effects of Oxidizing Agent and Surfactants
In addition to activity and stability at high pH range and various temperatures, enzymes incorporated into detergent formulations must be compatible and stable with all commonly used detergent compounds like surfactants, bleaches and other additives which might be present in the formulation (stability during storage and washing)
![]()
Table 1. Effects of various enzyme inhibitors and metal ions on the activity of the crude alkaline enzyme from A. arilaitensis Re117.
The crude enzyme was pre-incubated with various enzyme inhibitors for 30 min at 25˚C and the remaining activity was determined at pH 9.0 and 50˚C. Enzyme activity measured in the absence of any inhibitor was taken as 100%. The effect of metal ions on the activity of the crude enzyme was determined by incubating the enzyme in the presence of various metal ions for 15 min at 50˚C and pH 9.0.
[3] . Important commercial detergent proteases like Subtilisin Carlsberg, Subtilisin BPN, Alcalase, Esperase and Savinase are stable in the presence of various detergent components; however, most of them are unstable in the presence of oxidant agents [27] . Thus, it is desirable to search for new proteases with novel properties from as many different sources as possible. The A. arilaitensis enzyme preparation was incubated 60 min at 30˚C in the presence of additives and then the residual protease activity was assayed at pH 9.0 and at 50˚C (Table 2).
The crude protease was highly stable in the presence of the non-ionic surfactants. It retained 80 and 79% of its initial activity in the presence of 1% Triton X-100 and Tween 80, respectively. In addition, the purified enzyme was slightly affected by oxidizing agents, and it retained more than 70% of its activity after incubation in the presence of H2O2. The Arthrobacter crude enzyme activity increased by 118% in the presence of 0.1% (w/v) sodium perborate compared to the control test.
The high stability of the enzyme in the presence of oxidising agents is a very important characteristic for its eventual use in detergent formulations.
Stability of the crude enzyme of A. arilaitensis in the presence of various commercial solid detergents.
The high activity and stability of the crude enzyme of the A. arilaitensis preparation in the pH range from 7.0 to 11.0, and its relative stability towards surfactants and oxidizing agents are very useful for its potential application as detergent additive. To check the compatibility of the crude protease with liquid and solid detergents, enzyme preparation was preincubated in the presence of various commercial laundry detergents for 1 h at different temperatures. Commercial protease Purafect® 2000 E was used under the same conditions as the crude enzyme.
The data presented in Figure 9 show that the crude enzyme of A. arilaitensis Re117 is extremely stable in the presence of all solid detergents tested at 30˚C and 40˚C. It retained 100% of its initial activity in the presence of Axion and 98% and 78% in the presence of Ariel and Nadhif, respectively after 1 h incubation at 30˚C. A. arilaitensis proteases also showed excellent stability and compatibility at 40˚C with most of the detergents tested. The crude extract retained about 91% and 61% of its initial activity in the presence of Ariel and Nadhif, respectively.
(a)
(b)
Figure 9. Stability of the crude enzyme from A. arilaitensis in the presence of various commercial solid (a) liquid (b) detergents. The compatibility with commercial solid (7 mg/ml, in tap water, pH 9.0) and liquid detergents (diluted 100-fold in tap water, pH 9.0) was determined by incubating crude enzyme with detergents for 1 h at different temperatures and the remaining enzyme activities were measured at pH 9.0 and 50˚C. Enzyme activity of control sample without any detergent, incubated under the similar conditions, was taken as 100%. Values are means of three independent experiments. Standard deviations were ±2.5%.
3.10. Effect of Organic Solvents on Enzyme Activity and Stability
The use of enzymes in organic media has been one of the novelties of catalysis in the last few years. One major concern in this regard has been their instability/low-activity in organic media, since proteases may be suitable for peptide and ester synthesis under non aqueous conditions [28] . The effects of various water-soluble organic solvents (50%, v/v) on the stability of the crude enzyme were investigated. Half-lives of the crude protease activities are shown in Table 3. In the absence of organic solvents, the half-life of A. arilaitensis proteases was approximately 5 days. In the presence of methanol, isopropanol, hexane and acetone the half-lives of A. arilaitensis proteases were about 4, 3, 3 and 7 days, respectively. Interestingly, the stability of the enzyme was highly enhanced by DMSO, DMF and diethyl ether. The half-lives of the enzyme in the presence of diethyl ether and DMF were 17 and 20 days, respectively, and that in the presence of DMSO was more than 28 days of incubation.
These results are similar to earlier reports showing increased protease stability in the presence of organic solvent. In fact, the half-life of the B. cereus SV1 protease was approximately 34 days in the absence of organic solvent. However, in the presence of ethyl acetate and DMF, the half-lives were 51 and 48 days, respectively
![]()
Table 2. Stability of A. arilaitensis Re117 crude enzyme in the presence of various surfactants and oxidizing agents.
The alkaline crude enzyme extract was pre-incubated with surfactants and oxidizing agents for 1 h at 30˚C, and the remaining activity was measured at pH 9.0 and 50˚C. The activity is expressed as a percentage of the activity level in the absence of additives.
![]()
Table 3. Half-lives of the activity of the A. arilaitensis Re117 protease in the presence of 50% (v/v) organic solvents.
The alkaline crude enzyme was pre-incubated with various organic solvents (50%; v/v; dimethyl sulfoxide (DMSO), methanol, diethyl ether, n,n-dimethylformamide (DMF), hexane, acetone and isopropanol) at 30˚C with shaking (150 rpm) for 30 days. Aliquots were withdrawn at desired time intervals to test the remaining activity.
[17] . El-Hadj Ali et al. [25] showed that the half-lives of L. mormyrus proteases were about 18 and 17 days in the presence of methanol and acetone, respectively. Interestingly, the stability of the crude protease was highly enhanced by DMSO, DMF, diethyl ether and hexane; the half-lives of the enzyme in the presence of these solvents were more than 30 days.
3.11. Enzymatic Deproteinization of Shrimp Wastes by Goby Proteases
Chitin in the exoskeleton of shrimp shells is closely associated with proteins. Therefore, deproteinization in chitin extraction process is crucial. Chemical treatment requires the use of HCl and NaOH, which can cause the deacetylation and depolymerization of chitin. Many reports have demonstrated the application of proteolytic microorganisms for the deproteinization of marine crustacean wastes to produce chitin [15] [29] [30] . To the best of our knowledge, there are no available reports on the enzymatic deproteinization of shrimp wastes by A. arilaitensis proteases. The crude protease was applied for deproteinization of shrimp waste to produce chitin.
Different E/S ratios (10, 20 and 30) were used to compare the deproteinization efficiency. As shown in Figure 10, the deproteinization rate with a ratio of 10 was 79% ± 1.8%. The percentage of protein removal reached about 83% ± 2.71% with E/S = 20. Beyond a ratio of 20, no significant increase in the deproteinization rate was
![]()
Figure 10. Effect of the E/S ratio on the deproteinization of shrimp waste.
observed. The deproteinization activity of A. arilaitensis crude proteases was better than many bacterial proteases reported in many previous studies [31] [32] . The fact that deproteinization cannot reach 100% may be explained by the non-accessibility of enzymes to some proteins protected by chitin.
The obtained results demonstrated that the protease produced by A. arilaitensis Re117 could be used effectively in the deproteinization of shrimp wastes. Furthermore, the application of proteases or proteolytic bacteria for the deproteinization would be a good solution for the environmental problems associated with crustacean processing.
4. Conclusion
The present study reports the characterization and evaluation of proteases from A. arilaitensis Re117 as detergent additive and in shrimp waste deproteinisation. The crude protease was active in a broad range of pH 6.0 - 11.0 and exhibited a high stability in the presence of oxidizing agent and various laundry solid and liquid detergents, which made it an ideal choice for applications in liquid detergent formulation. Additionally, the crude protease was found to be relatively effective in the deproteinization of shrimp waste powder. The protein removal with an E/S ratio of 20 was about 83%. It was also stable in the presence of organic solvents.
Acknowledgements
This work was funded by the Ministry of Higher Education and Scientific Research, Tunisia and Pr. Hafedh Bejaoui a language expert at the Sfax Faculty of Sciences.
NOTES
*Corresponding author.