Aminated (Cyclopropylmethyl)Phosphonates: Synthesis and Anti-Pancreatic Cancer Activity ()
1. Introduction
Organophosphorus compounds, distinct for their (C-P) chemical bond resistant to hydrolytic enzymatic degradation, such as by phospholipase D, are noteworthy natural products [1] - [7] . These compounds prominently feature in phosphonolipids, abundantly found in diverse sources including shellfish, plant seeds, protozoa, and bacteria [1] [2] [3] [8] [9] [10] [11] . Geological evidence has traced the presence of phosphonates on Earth back hundreds of millions of years [12] . However, the role of natural phosphonic compounds in living organisms was only confirmed in the latter half of the twentieth century when 2-aminoethylphosphonic acid was isolated from rumen protozoa by Horiguchii and Kandatsu [13] .
The fascination with phosphonate compounds has surged recently due to their broad spectrum of biological activities. Synthesized phosphonates have demonstrated significant potential, including antiviral and anticancer properties [14] [15] [16] [17] . For instance, organic compounds with phosphonate functional groups serve as antiviral agents and anticancer therapeutics [18] , and short peptides with aminophosphonic acids exhibit strong antihypertensive and anti-osteoporotic effects [19] . Phosphonopeptides, where a phosphonic acid or a similar group (phosphine or phosphinic) replaces a carboxyl group at the C-terminus or in the peptidyl side chain, or where a phosphonamidate or phosphinic acid mimics a peptide bond, act as inhibitors of crucial enzymes linked to various diseases. These compounds have shown promising physiological activities and have potential medical applications, including as anticancer agents, inhibitors of aminopeptidases, and as blockers of protein tyrosine phosphatase [20] .
Pancreatic cancer, recognized as one of the most aggressive forms of cancer, is responsible for about 7% of all cancer-related deaths. Often diagnosed in advanced stages, it has a high mortality rate. Early detection through imaging techniques, along with the identification of genetic mutations in KRAS, TP53, CDKN2A, SMAD4, and BRCA genes, can enhance treatability. Despite this, treating pancreatic cancer remains challenging. While surgery and radiation therapy are employed, chemotherapy remains the predominant treatment modality [21] [22] .
This work is an extension of our ongoing research on organophosphonate compounds and is devoted to the synthesis of aminated (cyclopropylmethyl)phosphonates, which demonstrated anti-pancreatic cancer activity.
2. Results and Discussion
Previously, we reported the synthesis of (cyclopropylmethyl)phosphonates 2 using 1-alkynylphosphonates 1 with the reagent system Cp2ZrCl2/2EtMgBr/2AlCl3 (Equation 1) [23] .

Equation 1. Synthesis of (cyclopropylmethyl)phosphonates 2.
Inspired by the wide range of biological applications of aminophosphonates, our team sought to enhance the biological activity of our (cyclopropylmethyl)phosphonates compound 2 by introducing an amino group. We anticipated that positioning the amine group in close proximity to both the cyclopropyl ring and the phosphonate moiety might enhance the biological activity of these molecules.
To achieve this, we aminated a representative class of (cyclopropylmethyl)phosphonates using Hünig’s base [24] . Our starting material, diethyl ((1-(3-chloropropyl)cyclopropyl)methyl)phosphonate 5, was synthesized from diethyl (5-chloropent-1-yn-1-yl)phosphonate 3 via a five-membered ring zirconacycle intermediate 4, as depicted in Scheme 1 [23] . Subsequently, reacting 1.2 equivalents of an amine with two equivalents of Hünig’s base diisopropylethylamine (DIPEA) in a solvent-free process yielded the target aminated products 6a-c with isolated yields ranging from (68% - 73%).
These novel aminated cyclopropyl-methylphosphonate compounds (Figure 1) were separated from the reaction mixture on silica gel column (93% ethyl acetate:
![]()
Scheme 1. Synthesis of aminated (cyclopropylmethyl)phosphonates 6.
![]()
Figure 1. Pancreatic activity of the tested compounds (2a, 5, 6a-c).
7% methanol) and were analyzed by GC/MS, NMR, and elemental analysis.
Furthermore, their biological activity was explored as anti pancreatic cancer cells system. Luckily, they were found to possess potent suppression of the cancer cells in the cell line as shown in Figure 1.
Diethyl ((1-propylcyclopropyl)methyl)phosphonate 2a and the chlorinated counterpart 5 were evaluated to ascertain the impact of the amine group on anticancer activity. Intriguingly, the data revealed that amine integration into the cyclopropyl framework notably boosted activity against pancreatic cancer cells. As depicted in Figure 1, while the aminated derivatives 6a and 6b displayed moderate efficacy in inhibiting pancreatic cell proliferation, diethyl (1-(4-(benzylamino)butyl)cyclopropyl)methylphosphonate 6c emerged as the most potent inhibitor, exhibiting significant cell suppression at low micromolar concentrations with an IC50 of approximately 45 µM.
In summary, the newly synthesized aminated (cyclopropylmethyl)phosphornates demonstrated promising anti-pancreatic cancer activity, particularly when a benzylamine group was incorporated into the cyclopropylmethylphosphonate framework. Motivated by these in vitro findings, we plan to extend our research to in vivo studies and to evaluate the efficacy of these compounds against other types of cancer cells.
3. Experimental
The 1H, 13C, and 31P NMR spectra were recorded from solutions in CDCl3 on a Varian Mercury 300 spectrometer at 300, 75.5, and 121.4 MHz, respectively; the chemical shifts were measured relative to TMS (1H, 13C) and H3PO4.
Pancreatic cell proliferation assay: Pancreatic cancer cell line (PANC-1) from (Sigma-Aldrich) has been used to study the effect of the synthesised drug combinations. At regular basis cells were cultured in 25 ml culture flasks containing DMEM media (Sigma-Aldrich) plus (2 mM Glutamine, 10% Foetal Bovine Serum (FBS), and 1% pencillin/streptomycin solution) and kept at 37˚C CO2 incubator. Cells were counted and adjusted to a concentration of ~2 × 105/ml in complete culture medium, then (aliquot of 100 μl of cells) were seeded in 96-well sterial culture plate. This was followed by the addition of 50 μl of freshly prepared drug dilutions (from 10 mM to 100 mM) to the seeded pancreatic cells, drug free media was added as a control. Diluted drugs were added to the seeded pancreatic cells in triplicate, then plates were incubated for 24 hours at 5% CO2 and 37˚C. Cell viability was measured using Almar Blue (Bio-Rad cat No. BUF012A), for this purpose Almar blue (10% of final volume, 25 μl/well) was added to each well, followed by incubation at 5% CO2 and 37˚C for 4 hours. Fluorescence was measured at 535/590nm excitation/emission wavelength on plate reader. Cell viability percentages were calculated relative to untreated control.
General procedure for the synthesis of Diethyl (1-(3-chloropropyl)-cyclopropyl)methylphosphonate 5: To 0.306 g (1.05 mmol) of zirconocene dichloride dissolved in 7 ml of dry THF at −78˚C was added 1.05 ml of 2 M EtMgBr (2.1 mmol) dropwise in a 25 ml round-bottom flask. After stirring for 5 min at −78˚C, 1 mmol ( 0.22 g ) of 6-chloro-diethyl hex-1-ynylphosphonate was added. The reaction was gradually warmed to 25˚C and stirred for 2 hr. The reaction was cooled to −30˚C and maintained at −30˚C - 40˚C while 2 equiv. of AlCl3 were added. The reaction was stirred for 1 hr at −30˚C and was worked up with dilute HCl. The product was extracted with diethyl ether (2 × 15 ml) and was separated on silica gel column (70% petroleum ether: 30% ethyl acetate).
1H NMR (300 MHz): δ 0.30 (m, 2H), 0.47 (m, 2H), 1.15 (t, 2H, JHH = 7.5 Hz), 1.30 (dt, 6H, JHH = 6.9, 4JPH = 0.2 Hz), 1.36 (m, 2H), 1.71 (d, 2H, 2JPH = 18.0 Hz), 3.52 (t, 2H, JHH = 7.5 Hz), 3.98 - 4.15 (m, 4H). 31P NMR (121.4 MHz): δ 31.92. 13C NMR (75.5 MHz): δ 12.7, 12.8, 15.0 (d, 2JPC = 4.3 Hz), 16.4 (d, 3JPC = 6.3 Hz), 17.9, 24.7, 32.4 (d, 1JPC = 140.1 Hz), 40.2, 41.0, 47.4, 61.3 (d, 2JPC = 6.6 Hz). MS m/z: 270 (0.3), 268 (1.0), 233 (70.6), 231 (66.7), 219 (28.4), 205 (44.1), 177 (46.0), 163 (46.0), 149 (63.7), 148 (40.2), 125 (38.2), 111 (100), 99 (92.2), 97 (92.2), 81 (91.8), 67 (60.8), 41 (68.6), 29 (53.9). Anal. Calcd for C11H22ClO3P: C, 49.17; H, 8.25; Cl, 13.19; P, 11.53. Found: C, 49.08; H, 8.31; Cl, 13.29; P, 11.43.
Synthesis of diethyl ((1-(3-(pentylamino)propyl)cyclopropyl)methyl) phosphonate 6a:
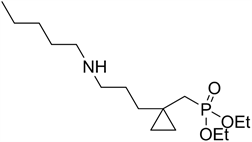
To 0.5 mmol of the chlorinated cyclopropyl product 5, 1.2 eqiv. of amyl amine and 2 eqiv. of Hunig’s base were added and the mixture was heated for 48 hours at 70˚C in a hermetically sealed 5 mL glass vial. Then the aminated cyclopropyl product was separated on silica gel column (93% ethyl acetate: 10 % methanol) to give the oily products in 73% yield.
1H NMR (300 MHz): δ 0.26 (m, 2H), 0.32 (m, 2H), 0.90 (t, 3H, JHH = 6.3), 1.20 - 1.45 (overlap, 10H), 1.30 (t, 6H, JHH = 7.2). 1.70 (d, 2H, 2JPH = 16.2 Hz), 2.80 (broad m, 2H), 2.90 (t, 2H, JHH = 6.3), 3.84 (q, 4H, JHH = 7.2). 31P NMR (121.4 MHz): δ 23.0. 13C NMR (75.5 MHz): δ 13.0, 13.2, 13.7, 16.7 (d, 2JPC = 6.6 Hz), 22.6, 24.1, 24.5, 25.4, 27.3, 28.7, 33.9 (d, 1JPC = 134.5 Hz), 35.8, 37.9, 39.6, 47.1, 59.6, (d, 2JPC = 5.1 Hz). MS m/z: 319 (5.8), 304 (18.8), 290 (23.2), 274 (40.3), 262 (45.8), 227 (65.2), 182 (100), 137 (29.4), 97 (43.2), 57 (87.3). Anal. Calcd for C16H34NO3P: C, 60.16; H, 10.73; N, 4.39; P, 9.70 Found C, 59.97; H, 10.62; N, 4.50; P, 9.79.
Synthesis of diethyl((1-(3-(isopropylamino)propyl)cyclopropyl)methyl) phosphonate 6b:
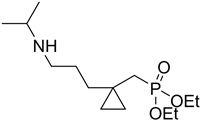
Identical procedure to the synthesis of 6a except adding ipropyl amine and the yield was 68%.
1H NMR (300 MHz): δ 0.29 (m, 2H), 0.35 (m, 2H), 1.20 (d, 6H, JHH = 7.2), 1.25 - 1.40 (overlap, 10H), 1.69 (d, 2H, 2JPH = 16.2 Hz), 2.62 (t, 2H, JHH = 6.3), 2.87 (m, 1H), 3.84 (q, 4H, JHH = 7.2). 31P NMR (121.4 MHz): δ 23.48. 13C NMR (75.5 MHz): δ 13.0, 13.6, 13.9, 16.8 (d, 2JPC = 6.6 Hz), 18.8, 20.3, 22.2, 27.5, 28.7, 28.9, 34.2 (d, 1JPC = 177.9 Hz), 41.2, 45.8, 60.0 (d, 2JPC = 5.1 Hz). MS m/z: 291 (7.8), 276 (12.2), 261 (18.8), 248 (55.7), 233 (60.4), 220 (49.8), 205 (23.2), 154 (61.8), 111 (78.1), 96 (20.3), 81 (60.5), 58 (53.2) 43 (100). Anal. Calcd for C14H30NO3P: C, 57.71; H, 10.38; N, 4.81; P, 10.63 Found: C, 57.65; H, 10.27; N, 4.94; P, 10.77.
Synthesis of diethyl (1-(4-(benzylamino)butyl)cyclopropyl) methylphosphonate 6c:
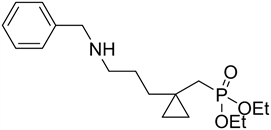
Identical procedure to 6a except adding benzyl amine and the yield was 70%.
1H NMR (300 MHz): δ 0.26 (m, 2H), 0.33 (m, 2H), 1.22 (t, 2H, JHH = 6.9), 1.28 - 1.38 (overlap, 8H), 1.59 (d, 2H, 2JPH = 16.8 Hz), 3.48 (t, 2H, JHH = 7.5 Hz), 3.71 (s, 2H), 3.95 (q, 4H, JHH = 6.9), 7.28-2.67 (overlap, 5H). 31P NMR (121.4 MHz): δ 22.75. 13C NMR (75.5 MHz): δ 13.0, 13.2, 13.4, 17.0 (d, 2JPC = 4.3 Hz), 21.8, 24.1, 24.5, 33.9 (d, 1JPC = 132.7 Hz), 35.1, 43.6, 50.4, 59.8 (d, 2JPC = 6.6 Hz), 127.9, 128.4, 128.7, 132.4, 131.7. MS m/z: 339 (8.7), 324 (17.2), 294 (40.1), 242 (51.2), 228 (74.0), 182 (55.8), 91 (100), 77 (78.5), 43 (47.2). Anal. Calcd for C18H30NO3P: C, 63.70; H, 8.91; N, 4.13; P, 9.13 Found: C, 63.58; H, 8.83; N, 4.24; P, 9.26.
2a: Diethyl ((1-propylcyclopropyl)methyl)phosphonate [23] .
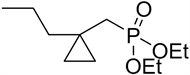