Soft Sediment Deformation Structures in the Sediment Deposits of Ganga River and Its Oxbow Lakes in Parts of Indo-Gangetic Plain: Implications for Quake Proxies and Paleoseismicity Tracking ()
1. Introduction
The Ganga basin is an important primary component of the Indo-Gangetic Plain which itself is the world’s largest repository of modern alluvial sedimentation in the Himalayan foreland. This foreland basin is the consequence of the collision of the Eurasian and Indian plates [1]. The basin deepened and evolved by sediment overloading and flexure of the lithosphere. It is bounded by the Indian Peninsula in the south and the Himalayan Mountains in the north. It is a huge and vast sink wherein alluvial sediment load is transported and deposited by three trunk rivers: Ganga, Brahmaputra, and Indus (Figure 2). Northward-drifted Indian plate when collided with the Eurasian plate (~50 Ma) ago [2]. The intervening Tethys Ocean closed and the generation of high-relief topography resulted. Since then, the Indian plate has locked its horn with the Eurasian plate and unabatedly tried to push it. And in the bargain, suffers under thrusting when accumulated stresses pulverize contact lithologies and brittle deformation results along the suture zone. It is suggested that the Himalayan orogeny has taken place in three main major pulses: early Miocene, late Miocene, and Pleistocene. By the end of the Miocene, a significantly thickened crust developed and the Himalayas attained their current height [3] [4]. Molassic sediments procured from the Himalayas got deposited into the foreland trough stretching from northeast India to northwest Pakistan [5] by rivers ancestral to the modern Indus, Ganges, Brahmaputra, and their large tributaries. Two more uplifting events of relatively lesser magnitude occurred in the post-Pleistocene period, which was responsible for river incisions [6].
Apart from these mega uplifting events, there have been numerous small-scale incidences of seismicity mainly due to the reactivation of thrusts or stress release mechanism [7] due to the sliding and thrusting of the Indian plate northward under the Eurasian plate at a rate of approximately 47 mm/year [8]. Available records of just the past ~ 700 years indicate the occurrence of 74 major and minor earthquakes and amongst which 71 occurred in past 50 years, whose magnitudes ranged between 3.5 to 8.6 [9]. From the geological point of view, the record of these quakes (if not all, then of at least majors) must have been preserved in the soft sediments under a suitable ambiance. The soft sediment deformation structures are thought to be potential paleoseismic archives [10]. Liquefaction, fluidization, and thixotropy are the major processes that cause a temporary reduction in sediment strength, resulting in the formation of soft-sediment deformation structures [11] [12] [13]. Shaking of water-saturated sediments by seismic shocks and factors controlling the physical characteristics of sediments such as porosity, permeability, cohesion generates soft-sediment deformation, and fluid escape structures [14]. Earlier research on SSDS was centered on older sediments, such as Proterozoic Vindhyan sediments, Chaibasa Formation, Cuddapah sediments [15] [16] [17] [18], Mesozoic sediments [19] [20], and Paleozoic [21] [22]. However, there is meager work on SSDS of Quaternary sediments of the Himalayan region, such as Karewas [23], and almost nil in the Ganga basin. As a result, a process-based understanding has been lacking since the evolution of the present landscape and its geomorphic diversity has not been incorporated into earlier studies. This research discusses the present state of knowledge of the Quaternary sediments in the Ganga basin, which contain SSDS with distinctive morphologies and has a persistent record of earthquakes during the Quaternary period.
Any earthquake of magnitude greater than five is considered to be capable of producing liquefaction-induced SSDS [24] [25]. The relationship between earthquake magnitude and distance of the formation and preservation of SSDS from the epicenter has been explored [25]. It has been suggested that an earthquake with a magnitude of 7 can cause liquefaction in an area 20 km away from the epicenter [25]. According to [26] [27], an earthquake with a magnitude greater than 8 can liquefy sediments up to 100 km away from the epicenter. However, the depth of the earthquake was disregarded in these studies, despite the fact that deep and shallow focal earthquakes have distinct impacts. Similarly, the existence of a specific climate in particular during the Holocene also controlled the formation of SSDS. For example, the Pleistocene glaciations were characterized by a series of glacial and interglacial episodes. These, in turn, had an impact on the fluvial regime and sediment production during different phases. The warm and humid climate is characterized as a precipitation period that would accelerate and enhance erosion in the catchments region leading to highly sediment-laden rivers, and consequently, the generation of thick sediment deposits whereas river discharges would be low with fine sediment load during the cold and dry glacial phases. The purpose of the present contribution is to define and classify the soft sediment deformation structures in the sediment deposits of the Ganga River and its Oxbow lakes in parts of the Indo-Gangetic plain and to discuss the importance of these structures which may serve as proxies of past seismic events with the futuristic application.
2. Study Area and Tectonic Setting
The Ganga basin constitutes the major part of the huge Indo-Gangetic alluvial plain. The Ganga basin represents the central portion of the Quaternary alluvial-filled Indo-Gangetic foreland basin system (Figure 1). The basin is restricted on the western and eastern sides by the NE-SW trending Delhi-Haridwar and Monghyr-Saharsa basement ridges, respectively [28] [29] [30] (Figure 2). The northern limit of the Ganga basin is defined by the Himalayan Frontal Thrust (HFT) [29] [31]. Whereas the southern limit is dispersed and represented by the highlands of the peninsular craton. Several workers have attempted to interpret the basement structure of the Ganga basin and the nature of sediment fillings, through geophysical surveys and deep drilling exploration for oil and groundwater conducted by the Oil and Natural Gas Corporation of India and Central Ground Water Board of India. A basement comprising Archean granites and Proterozoic metamorphosed supracrustal (collectively referred as Bundelkhand massif) has been established [32] [33].
Many subsurface faults run across the basement of the Ganga basin, including the Moradabad fault, Lucknow fault, Bareilly fault, Patna fault, and Malda fault [28] [34] (Figure 2). Because of the presence of several active basement faults, the thickness of the sediments in the Ganga basin varies significantly (2002) [35] [36]. Fluvial processes and tectonic activity are the prime controls of sedimentation in the Ganga basin. The Ganga basin is divided into four sections from north to south: Bhabhar, Tarai, Central alluvial plain, and Marginal alluvial plain. Bhabhar is a 10 - 30 Km wide gravelliferous sedimentary belt that runs
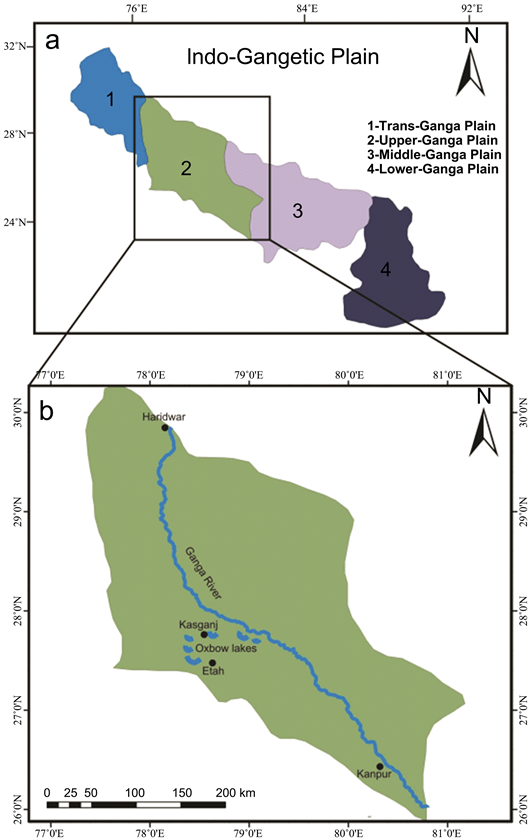
(i)
(ii)
Figure 1. (i) (a) The major divisions of the Ganga basin, in parts of the Indo-Gangetic (b) enlarged view of the upper Ganga basin in which study area lie also showing course of Ganga River, distribution of oxbow lakes and trenching at Kisaul Lake, Pachlana Lake and Daryaoganj Lake. (ii) Google map images of study area showing proximity of Ganga River and various lakes. Note all lakes are on the western side.
parallel to the Siwalik Himalayan range and has a steep slope. Next to Bhabhar, a 10 - 50 km wide low lying area is the Tarai belt, with extensive development of lakes, swaps, and small sandy rivers. The Tarai is a 10 - 30 km wide low-lying region adjacent to Bhabhar, with substantial growth of lakes, swamps, and smaller sandy rivers. The Central alluvial plain is dominantly a sand-mud deposit with some gravel and forms major part of the Ganga fill. The Marginal alluvial plain is a north-sloping terrain made up of sediments from the peninsular craton, with gravelly to coarse sandy rivers with entrenched meanders. The study area lies in the Upper Ganga plain. The area is selected for its uniqueness of harbouring numerous oxbow lakes in the vicinity of Ganga River on its western side, namely Daryaoganj Lake, Kisaul Lake, and Pachlana Lake (Figure 1(ii)). Hence an integrated analysis of quake impacts simultaneously on the soft sediment deposits of river and lakes can be done.
![]()
Figure 2. Map depicting the tectonic characteristics of the basement of the Ganga basin (compiled from Sastri et al. (1971), Rao (1973), Karunakaran and Ranga Rao (1979), Singh (2004) and Sinha (2005). Index: HFT: Himalayan Frontal Thrust, DHR: Delhi-Hardwar Ridge, FR: Faizabad Ridge, MSR: Monghyr-Saharsa Ridge. 1. Moradabad Fault; 2. Great Boundary Fault; 3. Lucknow Fault; 4. West Patna Fault; 5. East Patna Fault; 6. Monghyr-Saharsa Ridge Fault; R: River. Rectangle depicting study area.
3. Methodology
The Ganga River deposits sediments all along its floor and banks. Since it is meandering river, it is continuously changing its course in the northern direction from its position. This is evident by the presence of numerous oxbow lakes in the distal to proximal region in the south. Therefore, the sediment deposits in the flood plain region are quite younger than the oxbow lake sediments. In order to study sediment structures, appropriate sites were selected on the basis of the thickness, exposure and variation in sediment types after lengthy and rigorous survey. A trowel was used to flatten the faces of the sediment deposits at the selected locales. Wherever direct face was not available, ditches and trenches were excavated with the spade and the surface was smoothed. The uncovered surface is then left for drying for some time so that the sediment structures become sharp with blowing winds and thus can be viewed more clearly. The structures were photographed in situ using a high-quality camera (Canon EOS 1000D).
4. Distribution and Occurrence of Soft Sediment Deformation Structures
Numerous structures have been observed in both types of sediment deposits. It is interesting to note that there is hardly any overlap of structures in the river and lake deposits. Furthermore, the lake deposits are rich in structures compared to river deposits. It clearly suggests that paleoearthquakes were of higher magnitude than to recent quakes. A detailed account of these structures is given in the following paragraphs.
4.1. Clastic Dykes
Two sand dykes intruding into the overlying mud layer have been observed in the Ganga River deposits on its eastern bank at Anoopshahar (Figure 3). The larger dyke is 6 cm long and 1.5 cm thick, while the smaller one is 5 cm long and 1.3 cm thick. The larger dyke is bifurcated at its top (Figure 3(a)). Both dykes are inclined towards the south in the current direction of the Ganga River flow. The upward movement (intrusion) of sand into the mud layer is evident from the nature of these dykes as they are rooted in a basal sand bed. Sand dykes reported in the Dariyaoganj area are different both in dimensions and disposition than river deposits. A sand dyke reported from the Dariyaoganj area is significantly large (>12 inches high), which is broader at the base and becomes narrower upwardly with a rounded top (Figure 4). The dyke looks like a sub conical hill with steep and gentle slopes on opposing sides. In another trench of lake deposits in the same area, two small dykes of silty sand of 3 cm and 5 cm are found intruding into the overlying mud layer. It is pertinent to mention here
![]()
Figure 3. (a) Alternate sand and mud layers in Ganga River deposits at Anoopshahar. (b) Close up view of sand dyke bifurcated at top and inclined southward. (c) Sketch of bifurcated sand dyke shown in (b). (d) Photograph of alternate sand and mud layers in Ganga River deposits 10 m away from the previous location. (e) Close up view of sand dyke inclined southward. (f) Sketch of sand dyke shown in (e). Index: Yellow color—mud, Blue color—sand.
![]()
Figure 4. Sand dyke intruding into a mud layer in Daryaoganj Lake deposits (a). Close-up view of sand dyke shown in (b). Sketched view of sand dyke shown in (c). Index: Yellow color—mud, Blue color—sand.
that the tops of these dykes are bent in the opposite direction. Dykes are discordant sub vertical sheets/tabular bodies of clastic sediment which accumulate either “passively” by deposition into pre-existing fissures or “dynamically” by fracturing the country rock and injection of plastic material in response to pressure build up [37]. When there is significant density in turn permeability contrast in the coexisting sediment layers, sediments from the underlying layer (more permeable) flow into the overlying cap layer during seismicity induced fluidization [38]. According to [39], the clastic dykes are formed perpendicular to the propagation direction of earthquake waves. The injection dyke is one of the most convincing evidences of seismic shaking caused by tremors of magnitude of M > 6.5 earthquakes [40]. Width of the dyke may range from several millimeters up to several meters. The thickness of the dyke is considered a function of distance from the epicenter, i.e. a thicker dyke is proximal to the epicenter.
4.2. Clastic Sills
Horizontal inclusion of sand into mud layer is found in the lake deposits at Dariyaoganj area. These are the horizontal concordant injections of sediments, internally structure-less, set in contrasting sediment habitat. Such clastic sills are associated with lateral sand flow which may cause bending and shuttering of sediment. Such layer deformation is caused by liquefaction of the underlying source-beds. There are three large sills in the same deposit with different geometries and also some small associated sills. The contact between sand and mud layer is not regular but it is irregular forming sinusoidal contact. The uppermost clastic sill can be seen bending upward convexly in the right portion while convex downward in the left portion thus forming sinuosity (Figure 5). The middle clastic sill is also near sinusoidal but possesses deeper concave profiles. The lowest sill disposes nearly smooth geometry throughout its extent. These structures are developed by the intrusion of fluidized sands [41] which has been deduced an outcome credited to the liquefaction triggered by seismic shocks. The liquefaction is deciphered as a consequence of water-saturated material with high pore water pressure moving upward [41] [42]. Consequently, sills are connected with horizontal movement of fluidized sand into mud layer which also show upward and downward bending with shuttered behavior.
![]()
Figure 5. Clastic sills in the Lake deposits of Daryaoganj area. Index: Yellow color—mud, Blue color—sand.
4.3. Sedimentary Breccia
Brecciation is observed in lake sediments from the Dariyaoganj area. The unassorted angular to sub-angular fragments of mud of varying dimensions (2 cm to 8 cm) are found embedded in the lower layer of fine sand. These mud fragments appear to be floating in the fluidized sand (Figure 6). Disturbance caused by liquefaction results in fracturing, fragmentation, and collapse of the overlying brittle mud layer. These fragments, being heavier than the fluidized substratum, sink down. Brecciation resulting as a consequence of seismic activity can generate two types of breccias: autoclastic breccia and intraclastic parabreccia [43]. The initial break down of the brittle layer under the influence of a mild earthquake produces autoclastic breccia. However, intense quakes with cyclic aftershock tremors cause further displacement of pre-existing autoclastic breccia or fragment the brittle mud layer. These fragments sink down into the underlying, less viscous sand layer and produce intraclastic breccia. The observed structure may be termed intraclastic parabreccia because of the large variation in the shape and size of mud grains and their highly dispersed nature within the underlying sand layer, which indicates an intense paleoseismic event.
4.4. Ball and Pillow Structures
Ball and pillow structures are observed in the lake deposits in Dariyaoganj area. A ball structure with 6cm long axis and 3.5 cm short axis of silty sand with concentric rings is found in the mud layer (Figure 7(b)). The original layering parallel to the basal surface have been deformed to form concentric layering of the ball structure which show concave upward morphology similar to a small synform.
![]()
Figure 6. (a) Seismic mud breccia in the Lake deposits from Daryaoganj. (b-c) Enlarged views of Figure (a) to highlight spatial distribution of mud fragments within silty sand bed. For a better depiction mud fragments are filled with yellow color in (c).
![]()
Figure 7. (a) Deformed layers of fine sand and silt near Narora along the bank of Ganga River. (b) A close-up view of a concentric ball. (Note the presence of a sand tongue in the centre of the pillow structure; this could be the original layering that was once parallel to the overlying bedding.) Rupee coin is for scale.
The top surface of the structure is truncated due to erosion indicating that the ball structure was formed close to the depositional surface. The observed concentric ball structure has completely lost its contact with the overlying bed and now can be seen floating in the host layer of silty sand. The sediments both within and outside the ball structure is of similar grain size that is silt size. The concentric now deformed layers of the ball structures were initially parallel to the overlying and underlying beds. Similar deformational structures, typically displaying a ball-and pillow shape, have been observed by [14] [41] [44] [45] [46].
4.5. Dish and Pillar Structure
Dish and pillar structures of mud are observed from the lake deposits of the Dariyaoganj area. A mud pillar nearly 12 cm long is found intruding into the overlying sand layer. Within the same mud horizon, dish structures separated by pillar structures are found (Figure 8). Dish structures are concave upward soft sediments formed by the upward movement of pore water, which causes upward bending of sediments on both sides. Sediments structures are separated by pillars that reflect local upward movement of pore water, which causes upward bending of sediment at both sides [47]. Some workers attribute the formation of these structures to non-seismic mechanisms such as over-loading and sediment gravity flows [11] [48] [49] others associate them with seismic shakings [50].
4.6. Sudden Abutting of Mud Streaks
At places in the lake deposits of Daryaoganj, the continuity of mud and sand layers (streak) is found to be abruptly truncated, producing a nearly perpendicular plane (Figure 9). The alternate sand and clay streaks vary in thickness from 1 cm to 5 cm in the sand deposits. This structure generally occurs in association with the sedimentary breccia and at the same place. Sedimentary breccia is considered to be the unequivocal evidence of seismic activity, which indicates the formation of such truncated vertical plains as a consequence of seismic activity.
![]()
Figure 8. (a) Dish and pillar structures in the lake deposits of Daryaoganj Lake. (b) Close up view of dish and pillar structures. (c) Sketch of dish and pillar structures. Index: Yellow color—sand, Blue color—mud.
![]()
Figure 9. (a) Depicting the abrupt truncation of mud layers at Daryaoganj Lake. (b) Close up view of the abrupt truncation of mud layers.
4.7. Slump Folds
In our study area, we find slump folds as shown in (Figure 10(b)) where an overlying sand layer slumped downward and a thin mud layer just below the sand layer deformed in response, forming folds that are apparently monoclinal in orientation. It is suggested that such structures are formed when dense or less dense material abruptly slides over less dense (similar to creep folds) material downward under the influence of gravity.
4.8. Loadcast and Associated Flame Structures
Simple loads are the common soft sediment structures which are formed after the deposition of sediments. Load structures are generally formed as a result of density contrast between the overlying and the underlying layers of soft sediments at their interface. When the density of overlying layer is greater than density of lower layer, the possibility of formation of such structures becomes high. Flame structures are usually common associates of load structures. Loadcasts and associated flame structures in lake sediments are observed form Dariyaoganj area. The flame of mud of >2 cm height is found intruding into overlying sand layer. Similar structures but with different dimensions have been found at different location. The loadcast and associated flame structures are produced at the interface of two layers of contrasting density e.g. sand-mud layers, sand-silt layers where sediments of overlying layer sag or penetrate down into the lower
![]()
Figure 10. (a) Liquefaction and fluidization structures developed in cohesive mud layer and non-cohesive sand layer at Daryaoganj Lake. (b) Close up view of slumping structure results in formation of slump fold.
layer of differing density. The sediments of overlying layer sink down making the sediments of underlying layer intrude upward into the overlying layer forming flame structures. The formation of load and flame structures in soft sediments in a low or zero shear resistance situations is induced by liquefaction and fluidization in reverse density gradient systems [11] [49] [51] [52] and/or due to differential loading in Figure 11. In fluvio-lacustrine deposits where denser layer (sand layer) overlies, the less dense layer (mud layer) partial liquefaction and thixotropy may cause reduction in the strength of sediments that may lead to gravitational instability. These processes reduce the shear strength of sediments and sediments readjust according to their density. The high density sediments sink down and the underlying low density sediments move upward and thus produce load and associated flame structure. The resultant deformational structures are the consequences of reverse density stratification in sediments and largely controlled by dynamic viscosity (k) contrast of sediments. Structures associated with both the reverse and normal density gradient system are observed in Dariyaoganj area. The overlying sand layer is found penetrating the lower less dense mud layer forming load cast and associated flame structures (Figure 12(b)). Although these structures can be formed by gravitational instability, if they are found associated with typical seismites, they are usually thought to have a seismic origin. However, if the upper layer is less dense or of nearly the same grain size uneven loading seems to be the most reasonable cause for the development of load structures [53].
4.9. Uneven Interface of Two Contrasting Lithologies Structures and Deformed Mud Layer
Some uneven structures in the field are observed at the interface of sand and silty sand layers where the bottom of layer is highly irregular and the top of the same layer is sharp and planner (Figure 13) show a silty sand layer sandwiched between overlying mud and underlying sand layers. The contact between sand and silty sand layers is significantly irregular. Moreover, few flame like intrusion of lower sand layer into the overlying silty sand layer are also observed. On the other hand, the contact between the middle sand layer and overlying mud layer
![]()
Figure 11. (a) Load and associated flame structures in normal density gradient system in the Daryaoganj Lake. (b) Sketch of load and associated flame structures Index: Yellow color—mud, Blue color—sand.
![]()
Figure 12. (a) Liquefaction and fluidization structures developed in cohesive mud layer and non-cohesive sand layer at Daryaoganj Lake. (b) Close up view of simple load of sand and associated flames of mud. (c) Sketch of Photograph (b). (e) The sketch of photograph. (d) Another close up view of simple load and associated flame structures. Index: Yellow color—mud, Blue color—sand.
![]()
Figure 13. (a) Alternate layers of sand and mud, showing deformation structures at Anoopshahar along the bank of Ganga River. (b) A close-up view of a deformed mud layer bending upward. (c) Close-up showing an uneven interface of between two contrasting lithologies structures. (d) A closer look at the deformed sand and mud layers.
is abruptly sharp and planner. If sedimentation processes are related to traction currents with deposition of relatively thin layers of sediment and the top of the deformed structures are eroded, indicating that the formation of these structures occurred before the deposition of the overlying layers then the rapid load of sediments as a cause of liquefaction is unlikely and the SSDS produced are of seismic origin [14]. Similar structures are observed in the study area. This supports the origin of formation of SSDS in the area is seismic shocks.
5. Discussion
Liquefaction features are mainly generated by the tremors of earthquakes [24] [54] [55] [56] [57] [58] mentioned that the earthquake of magnitude more than 5 can produce liquefaction features. In our study area we found these structures well developed which validate the statement that the magnitude of paleo-earthquakes affecting the area should be at least of 5M. The identified SSDS suggests that area have been under continuous impact of quakes. Nature and type of structures confirm the active role of liquefaction, fluidization and thixotropy processes [13]. It further suggest that magnitude of earthquake was more than 5, because quakes of M ≥ 5 can generate SSDS in unconsolidated sediments lying in the close vicinity of epicenter [59]. Whereas earthquake of M > 7 can cause liquefaction in the region at around 20 km distance and quake of M > 8 up to 100 km from epicenter [25] [26] [27]. The area under study is hundreds of kilometer away from Himalaya. Available records of earthquakes indicate presence of plaeoepicenters at more than 100 kms from the study area. Furthermore, SSDS are better preserved in lake sediments than river sediments, indicate occurrence of high magnitude quakes in the geological past. It has been suggested that rivers of Indo-Gangetic Plain have continuously changed their courses. The distance between river and its oxbow lake is the measure of change in the course of river. The lakes of the study area are about 10 to 15 kms from Ganga River. It implies that after the formation of oxbow lakes, the area has suffered the impact of high-magnitude earthquakes. And that the region is under constant intimidation of seismic activity of high magnitude.
6. Conclusion
The present study reports the earthquake-induced deformation structures in sediments of the Daryaoganj Lake area of Kasganj district, Anoopshahar, and Narora town of Bulandshahar district located to the west of Ganga River in the western Gangatic plain. The origin of well-preserved liquefaction structures in the area seems to be the consequence of ancient earthquakes. The fluid escape structures are abundant in the study area. The appearance of these deformed structures is indicative of Himalayan tectonics and related seismic movement stretching out towards the southern peripheral Ganga plain. The soft-sediment deformation structures observed from the western Gangatic plain are proof of the advancement of the stress regime from the Himalayan front to the Indian Peninsula. All major earthquakes recorded so far from the middle Ganga plain have epicenters about 125 km away from the observed seismites location, which acknowledges the need for further research in similar tectono-sedimentary settings to revisit the influence of such seismic activities if previously underestimated. Earthquake activity in the Ganga basin is due to the collision of Eurasian plate and Indian plate. The seismically induced soft-sediment deformation structures in the Daryaoganj Lake, Narora Town. Anoopshahar is the indicator of this system. In addition to SSDS, deformed sediment layers are also been observed which suggest multiple episodes of earthquakes of differing magnitude.
Acknowledgements
The paper is part of the Ph.D. thesis of G.A. and M.S. The authors are thankful to the Chairperson, Department of Geology, AMU, Aligarh, for providing research facilities in the department. Financial assistance from University Grant Commission, New Delhi (UGC-MANF) to Gufran Ali is deeply acknowledged.