1. Introduction
In Physical Information Theory (PIT), mass, charge, radiation and vacuum are represented by three-dimensional structures with oscillator properties in four-dimensional fields and characterized by physical information. The structures are obtained from the Lagrange density and from the commutators of the communication relations of quantum mechanics via a Fourier transform [1] [2] under the conditions of the Hamilton principle [3] . Physical information is action, enclosed in a four-dimensional field; it characterizes the elementary objects, it is exchanged in interactions between the objects and it describes the change of the properties of the objects after the interaction. In contrast to quantum mechanics, in which an elementary object, e.g., an electron, is described by a wave function, the PIT distinguishes between the oscillators of the nucleus of the electron, which is described by scalar oscillators of mass and charge, and the electron shell, which is represented by photons of the static Maxwell fields. The electron nucleus and the electron shell consist each of a large number of oscillators. An interaction between two objects, such as between two electrons, then takes place in two stages: in an interaction between the photons of the photon clouds of both objects and an absorption and processing of the information in the scalar oscillators of the nucleus of the electron.
Up to now, oscillators for scalar fields and for the Maxwell fields have been constructed in the PIT. On the basis of the Hamilton principle, these describe the properties of elementary objects and their interactions in effects of quantum mechanics, for the phenomena of electromagnetism and in gravity on a four-dimensional correlation space; the effects are therefore causal and local. Details are referred to in the publications [3] .
All statements obtained with the help of the PIT are so far purely qualitative; the four-dimensional structures can only be quantified by computer simulation. Conclusions in the interpretation of the structures are qualitative in nature and are based on the assumption that action is generated by a μ = 0 oscillator in a four-dimensional vector field and that all operations occur under the conditions of the Hamilton principle. Only the elements required to understand the function of the mass oscillator will be discussed in more detail in this report: the μ = 0 oscillator that generates the action, the structure of the scalar oscillators and the Maxwell vacuum.
In PIT, the Maxwell vacuum is of central importance because it serves as a carrier and mediator of information in the interaction between elementary objects [4] . While processes in space-time are mainly described by real action, the Maxwell vacuum consists of a deactivated virtual part of the action and of an activated virtual part of the action. The activated virtual part of the action is gravity. Gravity consists of three contributions: the part formed at the Big Bang, the part caused by the gravity of the masses, and the portion of the action stored in matter due to the acceleration of the elementary objects during the Big Bang [5] . The Maxwell vacuum superimposes the photon cloud of the elementary objects [3] , and is thus part of the interaction of mass objects with their photon cloud.
Physical information theory offers alternative interpretations for the effects of quantum mechanics, electromagnetism and gravity. We want to show in this report that the action is stored in the form of activated virtual action of gravitons in the oscillators of the mass. This results in the following statements by means of the PIT:
→ Virtual action is stored in the mass oscillator: mass is stored virtual action.
→ Action is exchanged between photon cloud and mass oscillators.
→ Absorption/emission of action leads to changes in the rest frame.
→ There is a non-relativistic range below the non-relativistic limit (NRL) and a relativistic range above the NRL in the mass oscillators.
→ Above the NRL, additional mass is formed by absorption of action, detectable by increasing the action of gravitons in the photon cloud.
→ The inertia of the mass is caused by the stored action in the mass oscillators.
→ A change in the rest system is affected by changing the virtual action.
2. The Generator of Action
In the theory of physical information, the action is generated by a μ = 0 oscillator. Enclosed in a four-dimensional Maxwell field
, the action forms the physical information. The four modifications of the generator of the action are shown in Figure 1. The arrows between a creator
and an annihilator
describe correlations; the arrows are always directed from a creator to an annihilator. The μ = 0 generator of action is obtained from the four-dimensional commutators of quantum mechanics of the Maxwell fields [6] , and with the help of the fields of the trace of the energy-momentum tensor of the Maxwell fields [7] . These lead to four-dimensional structures of the photons of static Maxwell fields and to the structures of the dynamic Maxwell fields, i.e., the light. These structures should not be reproduced at this point for reasons of space, they are discussed in detail in [6] . Instead, we only look at the generator of action in Figure 1, which is part of the four-dimensional structures of the Maxwell fields.
The generator of the action (Figure 1), can be formed in four different versions. To explain this, we interpret the strings as currents that pass between a creator
(the source) and an annihilator
(the sink) via the correlations of the partial derivatives of the cube. Two currents that lead between a positive
and negative
creators via the cube to a negative
and positive
annihilator, and are each assigned to the two parts (1/2)
![]()
Figure 1. Different modifications of the action generator: (a) Deactivated vacuum; (b) Graviton; (c) For positive charge; (d) For negative charge.
and (0/3) of a Maxwell photon, generate units of action. In such a generator of action the currents can have different orbital directions. If the current from a positive creator
to a negative annihilator
has a positive circulation and the associated current from a negative creator
to a positive annihilator
a negative circulation (clockwise), then the resulting current and the real action generated by the two currents (in relation to the negative direction of circulation) is negative. If the current runs from
to
in the negative direction of circulation and the current from
to
in the positive direction of circulation, then positive real action is generated. If, on the other hand, both currents from
to
and
to
have the same direction of circulation, then both currents extinguish each other; no action is generated. In this case, we are talking about deactivated virtual action.
These statements can be verified by transforming the correlations characteristic of the commutators into space-time: negative and positive commutators of quantum mechanics are obtained by means of the horizontal correlations and in the case of the same circulation directions, the products belonging to the correlations erase each other [6] . The vertical correlations in the μ-unit cubes represent the products of the derivatives of the fields in the Lagrange density and in the equations of motion. The vertical correlations in the unit cubes describe the connection of the correlation structures with space-time: The current in the strings of the μ = 0, 1, 2, 3-unit cubes always lead from the creator plane to the annihilator plane of the correlation structure. This direction also represents the direction of motion of a Maxwell photon in space-time.
The four versions of the action generators shown in Figure 1 each consist of two commutators, which we simplistically refer to as photons O and X, because they are characteristic of the different spin of the O and X Maxwell photons. The Maxwell photons O and X consists of two parts (1/2) and (0/3); each of them generates one of the two currents between a creator and an annihilator. The photons O and X of the static Maxwell fields form O-X photons [8] ; they consist of a superposition of the two static photons O and X with different spin. At the unit cube μ = 0, they each occupy a corner for the incoming currents in the creator plane and a corner for the leaking currents in the annihilator plane of the structure of the photon. Thus, for the photons of the static Maxwell fields, four of the eight corners of a unit cube are occupied by one O-X photon. The other four corners occupy the photons O and X of the dynamic Maxwell fields, i.e., those of light.
Figure 1(a) shows the action generator of a photon of the Maxwell vacuum. This static photon of the Maxwell vacuum contains two photons O and X, whose action-generating currents
to
and
to
have the same direction of circulation and thus contain the deactivated virtual action. Figure 1(b) shows the action generator of the graviton. This consists of the two static photons O and X, each of which has the same real action but different signs of the action. A comparison of the two Figure 1(a) and Figure 1(b) shows that they are almost identical: the difference between the two is only in the circulation of the currents within the cube (which is indicated by different representations of the arrows in Figure 1). The Maxwell vacuum is formed by superimposing the generators with deactivated currents (Figure 1(a)), and with the activated currents of the gravitons (Figure 1(b)). Finally, Figure 1(c) shows the currents for the generation of positive action, i.e., positive charges, and Figure 1(d) shows the currents of negative action for negative charges.
3. Properties and Function of the Scalar Oscillator
The reports [3] [7] describes a scalar oscillator that interacts with the photons of the static Maxwell fields, exchanges physical information with other oscillators and thus describes the properties of objects and the interaction between objects. The scalar oscillator which describes the wave properties of particle or anti-particle is represented in an oscillation state Z2 for the μ = 0 oscillator in (1) (all fields are dimensionless).
μ = 0 oscillator, state Z2(1):
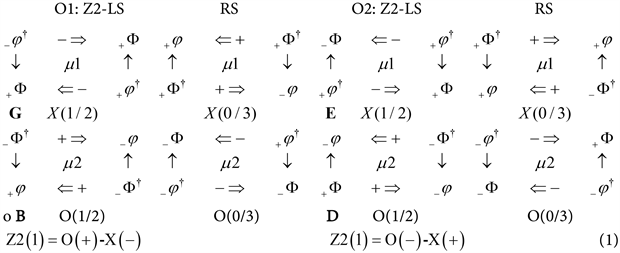
The scalar oscillator is first derived from the fields of the scalar Lagrange density and from the fields of the scalar four-dimensional commutators of the communication relations [7] . The scalar oscillator contains the correlations of the scalar fields and two oscillators O and X of the four-dimensional scalar commutators of the communication relations, which interact with the same scalar commutators O and X of the “scalar” photons of the photon cloud. It is then shown that under the conditions of wave properties, the scalar oscillator oscillates with the same four-dimensional commutators as the photons of the static Maxwell fields, represented by the vector potential. We identify the currents representing the “scalar photons” with the currents of the photons of static Maxwell fields. The oscillator (1) already contains the currents of the photons of the static Maxwell oscillators, instead of the “scalar photons”. The currents of the photons of the static Maxwell fields (1/2) and (0/3) are represented in (1) simplifying by double arrows with direction (negative circulation clockwise) and current sign. The signs in brackets describe the signs of action in the μ = 0 oscillator.
The scalar oscillators interacting with the photons of the static Maxwell fields always consist of two oscillators O1 and O2, which are conjugated complex. Each of the scalar oscillators O1 or O2 consists of four closed paths formed by the two currents (1/2) and (0/3) in the unit cubes (Figure 1), related to the static photons O and X. The currents of the absorbed photons O and X interact with the field-field correlations of the scalar fields, e.g., with
, where again the arrow represents the correlation between creator and annihilator.
In this report, we want to analyse the function of the scalar field-field correlations in (1), which represent the mass in the scalar oscillator (1). For this purpose, we construct a model consisting of the generators of the action (Figure 1) and will show that in this way the properties of the mass oscillators
of the scalar oscillators (1) can be simulated within the framework of the PIT. With the help of the mass oscillator designed in this way, we want to determine the desired statements about the mass oscillators as described at the end of the introduction.
4. Properties of the O-X-(O, X)-Oscillator
Figure 2 shows a model of a μ = 0 oscillator that can describe the properties of the field-field correlations
in the scalar oscillator (1) using the properties of the action. On the left in Figure 2 in A the mass oscillator
and in C the mass oscillator
is shown, on the right the action generators of the two O and X photons contained in (1). According to the illustration in (1), the two photons O and X, contained in (1), interact with the scalar field-field correlations
via correlative interactions. The scalar field-field correlations on the left in Figure 2 consist of a superposition of an O-X graviton (Figure 1(b)) and of two action generators of the dynamic photons O and X of light. The direction
![]()
Figure 2. Model of a matter-oscillator, expressed by μ = 0 oscillators of the vector potential.
of motion in space-time in a unit oscillator is described by the activated vertical correlations (two parallel arrows in Figure 1 and Figure 2), which correspond to the products of the derivatives in the equation of motion and describe the direction of motion in space-time. To simplify the discussion, we want to refer to the oscillator on the left in Figure 2 as an O-X-(O, X) oscillator, the generators of action for the photons of light as a dynamic part (dyn) and the gravitons as a static part (stat1) of the O-X(O,X) oscillator. The O-X-oscillators at right in Figure 2 we abbreviate by (stat2).
The direction of motion (two parallel vertical arrows) of the gravitons (stat1) in the O-X(O, X) oscillator is directed in the opposite direction to the direction of motion of the dynamic oscillators (dyn). With the same action in the static and in the dynamic oscillators of the O-X (O,X) oscillator an equilibrium is thereby formed in all rest frames. The two photons O and X (stat2) in the scalar oscillator (1) also form an O-X graviton. On the one hand the O-X-graviton interacts with the oscillator of the masses
and on the other hand with the gravitons in the photon cloud (not shown in Figure 2).
The real action in the static and in the dynamic part of the O-X-(O, X) oscillator is zero, because in the O and X photons of these parts the real action is the same and with opposite sign. The formation of the same action with different signs is characteristic of mass oscillators, because they are formed under the conditions of the Hamilton principle during the Big Bang [9] (principle of minimizing the action [5] ). The O-X-(O, X) oscillator in Figure 2 represents a product of scalar density
located to the photon O or X of the O1 oscillator (1) containing two such products:
and
that are assigned to the two conjugated photons O and X of the graviton (stat2). The oscillator O2 in the scalar oscillator (1) is conjugate complex to the oscillator O1 and contains, for example, the inverted signs of action and directions of the currents in the O-X(O, X) oscillators.
To describe an O-X-(O, X) oscillator in the context of the PIT as a mass oscillator, i.e., the products
or
, we assume that the static (stat1) and dynamic part (dyn) in the O-X (O, X) oscillator each contain the same fixed amount of the activated virtual action (Figure 1(b)), and whose action under non-relativistic conditions is greater than the action contained in the static O-X part (stat2) of (1) (right in Figure 2).
In the rest frame (no interaction) the static part (stat1) of the O-X-(O, X) oscillator, together with the static part of the photons O and X (stat2) in (1), with its content of the action is in equilibrium with the static O-X photons of the photon cloud (Figure 3(a)). The absorbed oscillators O and X in (1), (stat2) contain the action of the gravitons of the vacuum and the gravitons that characterize them in their rest frame. Characteristic of the rest frame with a relative speed is the content of action compared to other rest frames with a different relative speed. The vacuum contains the gravitons that were formed during the Big Bang and, by expanding the cosmos, contain the density of the action at the present time. The deactivated vacuum according to Figure 1(a) has no influence on the equilibrium and is only the carrier of the events in the universe [4] .
5. Interaction of Scalar Oscillators
In the PIT, the interaction between two elementary objects is described in the following steps [7] : By overlapping the photon clouds of two objects, physical information is exchanged in the form of deltas of the (four-dimensional) action under the condition of the Hamilton principle (Newton’s third law). Subsequently, the changed information (the deltas of action) is transferred to the oscillators O and X (stat2) absorbed in (1), whereby the action in the non-relativistic case in the O-X-(O, X) oscillator does not change, i.e., the absorbed information is stored in (stat2). The oscillator (1) oscillates at interaction in four oscillation stages, changing the amounts of the action of the absorbed photons O and X in (stat2) and changing its rest frame. If, in the non-relativistic case, action is added up as a result of the interaction, then the relative speed increases, if the action in the O and X photons is reduced, then the relative speed decreases [7] . The action in the static and dynamic part of the O-X(O, X) oscillator does not change, it is below the not relativistic limit (NRL) a measure of the mass of the oscillator. In the following compilation (2), the oscillator (1) is shown in a further simplified form.
(μ1) action change in μ = 0 of the X-photon:
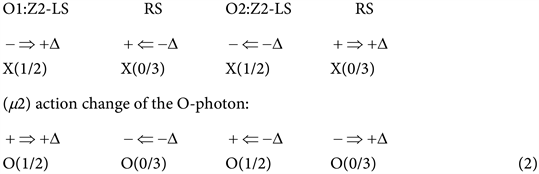
Only the currents of the oscillators O and X (stat2) and now additionally the currents of the deltas of the action are entered, after a complete change of the rest frame. For simplicity, the field-field correlations are omitted. Each four-dimensional delta of the action consists of the two currents (1/2) and (0/3) and can be written simplistically in the form
for a positive and
for a negative delta [3] ; the single deltas represent the currents (1/2) and (0/3) and the sum of both the positive or negative action (in space time the commutator of communication relations). In (2), the currents of the deltas are assigned to the currents of the photons O and X (stat2) in such a way that they have the same direction of circulation and can therefore be overlapped by superposition.
In (2) the common oscillation behaviour of the two oscillators O1 and O2 after a complete absorption of new information in the form of deltas is shown. Each delta of the action consists of two current parts, which have an amount, a direction and a sign and which are assigned to the two oscillator parts (1/2) and (0/3) and thus to the commutators of the communication relations. When the action is activated, the two current-components (1/2) and (0/3) have the same amount, always have opposite directions of circulation and opposite signs. When the delta of the action is absorbed in (stat2) of (1), under the conditions of minimization of the action, they are assigned to the part (1/2) or (0/3) of the oscillator O or X that has the same direction of circulation.
According to (2), in the O1 oscillator the deltas are added by the amount in O (0/3) and in O (1/2) to the O oscillator and from the X oscillator the deltas in X (1/2) and in X (0/3) are subtracted. Thus, in the case of an interaction in a gradient, the deltas of the action in O1 are added to the O-oscillator and subtracted from the X-oscillator. With the O2 oscillator, this is the other way around: the deltas are subtracted from the O-photon and added to the X-photon. In the following, the effect of this interaction on the behaviour of the O1 oscillator using the O-X (O, X) model oscillator is analysed.
The O-X-(O, X)-oscillator is with its static photons (stat1) in interaction with the gravitons (stat1) in the photon cloud, but not with its dynamic part (dyn), because the two photons O and X in (stat2) and the photons of the vacuum (stat1) have the structure of the static photons, while the structure of the portion (dyn) in the O-X-(O, X)-oscillator is not present in the photons O and X of (stat2) or in vacuum. The gravitons produced by the O-X-(O, X) oscillator in (stat1) are in balance with the gravitons of the vacuum (Figure 3(a)). The connection between the part (stat1) of the oscillator (1) with the gravitons in the photon cloud occurs under condition of the Hamilton principle by the opposite signs of the μ = 0 oscillator.
6. Interaction before and after the NRL
In a gradient of gravitation with increasing action, deltas of the action are absorbed with the same sign. For a simplifying discussion, we assume that the deltas also have equal amounts (homogeneous gradient). If we consider the state before the NRL (Figure 3(a)), then in one of the two photons (in O of O1 (stat2))
![]()
Figure 3. Schematic representation of the content of the virtual action in the scalar oscillator (left) and in the photon cloud (right) in the rest frame below in A and above the NRL in B.
the positive action increases and (in X of O2 (stat2)) the negative action increases in (1) according to (2), wherein assuming the same delta, the positive and negative action between the two scalar oscillators O1 and O2 are delete each other (in O1 deltas are added to positive O and in O2 negative delta are added to negative X), so that the real action is zero and only the virtual action increases. In the other part of photons (in X of O1 (stat2) and in O of O2 (stat2)) in (1) according to (2), the addition of the deltas leads to a decrease in the currents because the currents of the deltas are subtracted. In this acceleration phase (Figure 3(a)), as a result of the absorption of the deltas in the O-photon of O1 and in the X-photon of O2, the proportion of the virtual action in the scalar oscillators (1) increases with the values in (stat2). We interpret this increase of action with an acceleration (change of currents in the vertical correlations [3] [7] ) by absorption of virtual action from the rising gradient in the two parts (stat2). As discussed below, the simultaneous subtraction of the currents of the deltas in the X-photon of O1 and in the O-photon of O2 results in an increase in the action in the photon cloud. During a complete four state interaction process the same action is absorbed in the oscillator (stat2) with a change of the rest frame and a change of action in the gravitons of the photon cloud (stat2) (Figure 3(a)).
By continuous supply of action in a potential gradient with increasing action, the NRL is achieved when in the oscillator (1) according to (2) in the two parts of the oscillators (stat1) and (stat2), equal values of the action in the absorbed state and in the photon-cloud are achieved, which corresponds in Figure 3(a) by equally high values for the action in (stat1) and (stat2). The behavior of the oscillator (1) above the NRL is shown in Figure 3(b). Here it is assumed that a new rest frame in the area of relativistic speed already exists. The equilibrium between the static photons in the oscillator (1) and in the photon cloud is determined by the total supplied action in (stat1) and in (stat2), which is the same on both sides-on the oscillator (1) and in the photon cloud. The equilibrium between the action in the photon cloud and in the oscillator (1) forces, under conditions of the Hamilton principle, action with equal parts in (stat1) and (dyn) of the O-X-(O, X) oscillator. The increase in the action in the O-X (O, X) oscillator beyond the value of the non-relativistic case (Figure 3(a)) corresponds to an increase in mass. This is visible above the NRL in the equilibrium between the action of the oscillator (1) and the action of the photon cloud (Figure 3(b)). Additional action is stored in both parts, due to the different oscillation mechanism of the O-X-(O, X)-oscillator, as will be discussed with Figure 4 in next section. The concentration of the action in the photon cloud forces the equality of the action in the absorbed state and in the photon cloud, and the proportion of the action absorbed in the O-X (O, X) oscillator also contributes to this equilibrium of action in gravitons.
7. Information Storage in Mass Oscillators
Individually, the μ = 0 generators of the static and the dynamic photons O and X oscillate by flowing currents from two creators with different signs in the creator plane of the correlation structure diagonally over the cube to two annihilators with opposite signs in the annihilator plane. In this way, an activated photon forms a complete orbit in the cube (Figures 1(b)-(d)), which we can also interpret as the actual process of forming action [3] . O and X static or dynamic photons have only two of the four vertical correlations in common (two parallel vertical arrows). We interpret the circuits, formed by two strings, as currents that together form during a change of state the action.
In the O-X-(O, X) oscillator, on the other hand, two static and two dynamic photons superimpose with different spins (O, X) in such a way that eight of twelve correlations are activated in the cube. This becomes visible when we determine the signs of the partial derivatives at the corners of the cube. As the currents of the static and dynamic photons overlap, the signs of these partial derivatives change, which can be regarded as infinitesimal changes of creators and annihilators. While these signs partially neutralize each other in the static or dynamic O and X photons, all corners receive new signs when the O-X-(O, X) oscillator is formed, as they are reproduced in Figure 4. Thus, the orbits in the cubes of the mass oscillator change by oscillating now in two opposite orbits in each cube in two states on the opposite sides of the cube, while the remaining four correlations are deactivated (no current flows between fields of the same sign). O and X have each activated other opposite planes by circulating currents.
The result of Figure 4 can be inserted into the statement of the scalar oscillator (1). Each of the two closed paths of an oscillator O or X in (1) includes an oscillator (Figure 2), i.e., a
or
product. This is shown for the oscillator O1 for two oscillation states in Figure 4. In contrast to the representation of the scalar oscillator in (1), according to the development of the oscillator in Figure 2, the orbits in Figure 4 are now three-dimensional, which cannot be realized in the one-dimensional scalar oscillator. The three-dimensional representation corresponds to the spatial connection of the scalar μ-oscillators. The second state Z2 of the O1 oscillator, as well as for (1), is indicated by the fact that all correlations are reversed, which corresponds to a reversal of the signs of the μ = 0 oscillator. The oscillator O2 emerges from the oscillator O1 by reversing all correlation directions. According to (1), each photon O or X is to be assigned a field-field product: in O1 the photon O in Figure 4 is related to the product
and the photon X to the product
. Accordingly in state Z2 the O-photon relates to
and the X-photon to
.
Figure 4 describes the oscillation behaviour of the O-X(O, X) oscillator below the NRL. It contains in (A) for the O1 oscillator the linking of the X-photon from (stat2) with the O-X(O, X) oscillator in state Z1 and in (B) in state Z2. In (C) is the link of the O-photon from (stat2) to the O-X(O, X) oscillator in state Z1 and in (D) in state Z2. It thus describes the oscillation behaviour of the O1 oscillator between the O-X (O, X) oscillator with the two photons O and X of the (stat2)-region. The photon cloud is not included in this representation.
If above NRL the proportion of the action in the photon cloud increases above the value of the action of the O-X(O, X) oscillator, then in O1 the X-photon of (stat2) supplies the static part (stat1) with higher currents in both states and the O-photon of (stat2) in both states supplies the (dyn)-part with higher currents. Since O2 is conjugated complex, in O2 there is an increase in the currents in the static part by the O-photon and in the dynamic part by the X-photon. Temporarily, in a rest frame above the NRL, the effect in the O-X-(O, X) oscillators will increase the action in the field-field correlations, which is associated with an increase in mass, whereby the non-relativistic rest mass does not change (schematic representation in Figure 3(b)). Simultaneously with the increase of the action in the O-X-(O, X) oscillators, as discussed, the action in the photon cloud increases, so that in the equilibrium between oscillator (1) and its photon cloud, the same amount of action is present. While the mass, represented by the O-X(O, X) oscillator, remains unchanged below and above the NRL, because it is stabilized simultaneously by the currents of both photons O and X of both parts (stat1) and (dyn), the relativistic mass is only temporary, because it only records an increase in action either in the static part (stat1) of O1 and in the dynamic part (dyn) of O2, or in static part of O2 and in dynamic part of O1; it is not forming a stable configuration and disappears again when the speed of the object below the NRL is reduced.
The different oscillation of the mass oscillators makes up the stability of the field-field oscillator. The storage of the action absorbed by interaction thus takes place under common oscillation of all components of the scalar oscillator (1) and the different orbits and signs of the currents of action in the unit oscillator μ = 0 allow the formation of a rest frame with an equilibrium of the action between the content of action in the scalar oscillator and the action stored in the gravitons of the photon cloud.
![]()
Figure 4. Oscillation of the O-X-(O, X) oscillator in the scalar μ= 0 oscillator O1 under interaction with the absorbed photons O and X.
8. Inertia of the Mass and Speed of Light
When discussing the O-X (O, X) oscillator, we have already assumed that the change in action will result in a change in the rest frame. This section will examine this issue in more detail. From this assumption following effects should follow: If the action in the oscillator (1) increases, then the relative speed increases and approaches the speed of light at high amounts of action (energy). If the action in the O-X (O, X) oscillator decreases, then the oscillator (1) takes on the properties of photons or gravitons and the relative velocity approaches the speed of light. The supply of action to the oscillator is associated with inertia and the question is which mechanism causes this.
For the following discussion of a matter oscillator (1), we simplistically assume that it is located in a rest frame, in which there is equilibrium of both the virtual action and the oscillation directions between the oscillator (1) and the photon cloud. In this case, the oscillator is located in a homogeneous and isotropic environment and moves at its initial speed, which it received during its formation; this is characterized by the amount of the action in (stat2). Without their connection to the mass oscillator (1) the gravitons (stat1) and (stat2) in the photon cloud of (1) would move at the speed of light at the same rate as the photons of light; this occurs by inducing structures in a vacuum in one step in the direction of their vertical correlations, and in the next step their currents of action would move into these structures at the speed of light, [10] .
However, the locomotion of the photons of the photon cloud at the speed of light is prevented by their connection to the mass oscillator (1), which causes the inertia of the system, consisting of the mass oscillator and the associated photon cloud. The photon cloud is connected to the mass oscillator (1) and to the O-X (O, X) oscillator via the absorbed O-X photons (stat2). This connection is described schematically by Figure 4. Without the connection to (stat2), the O-X (O, X) oscillator is a static structure in which all currents are extinguished at any time. The oscillation of the gravitons of the photon cloud over (stat1) and (stat2), according to the representation in Figure 4, is imposed on this system. The system, consisting of the matter oscillator (1) and its photon cloud, oscillates in a rest frame with a uniform frequency, which is significantly determined by the O-X (O, X) oscillator. If the action in the photon cloud changes as a result of an interaction, then the oscillation frequency also changes; it becomes greater when the action increases and becomes less when the action is reduced.
The changes in the action in the oscillation system are forced by the change in the action in the photon cloud. A change in the action in the system of the “oscillator (1) “photon cloud” occurs under the conditions of the Hamilton principle. According to this principle, a change in the action causes a change in the currents in the oscillator system “Oscillator (1), photon cloud”. A change in currents requires the formation of a current of equal amount, same sign and opposite direction, i.e. a delta of action with the same amount and opposite sign. The formation of this induced delta is the reason for the inertia of the oscillator system.
The oscillators of the PIT are four-dimensional covariant, which corresponds to the conditions of Special Relativity Theory. In [11] it is shown that a change in the action in the oscillator (1) is associated with a change in the canonical momenta. On space-time, therefore, the relativistic mass is in relation to the relative velocity. On the other hand, the mass is related to the frequency according to the Compton relationship. Just as the relativistic mass depends on the relative velocity, so the oscillation frequency of the oscillator also depends on the relative velocity [11] :
, where
is the oscillation frequency at the rest frame of the mass and v is the relative velocity.
On the correlation space, the changes in currents in the vertical correlations occur in particle properties in the supply of real action (real delta in O or X) and in wave properties in the supply of virtual action (delta simultaneously in O and X) in the vertical correlations [7] . It follows that the action in the oscillator (1) determines the relative velocity and the change in the action causes a change in the rest frame. Since the increase in the action corresponds to the increase in energy, it can also be assumed that an increase in the action corresponds to an increase in speed.
According to the above discussion, the gravitons (stat1) and (stat2) in the photon cloud, unless bound to the oscillator, would move at the speed of light. Binding to the O-X (O, X) oscillator prevents this. If we think about the action in the O-X (O, X) oscillator gradually decreases, then we reduce the inertia of the system of oscillator (1) and its photon cloud. If we reduce the action in the O-X-(O, X) oscillator to a minimum amount, then the system “mass oscillator (1)-photon cloud” will move at almost the speed of light. It further has mass contained by the action bound in the O-X (O, X) oscillator, and is formed by the gravitons of the photon cloud. So, if we reduce the action in the O-X (O, X) oscillator, then the oscillator (1) takes on the properties of photons, or gravitons, and the relative velocity approaches the speed of light.
For the O-X-(O, X) oscillators there are three variants [11] : (a) the mass oscillator described in this report, which is formed from the two parts, the static (stat1) and the dynamic (dyn), wherein each of the two parts contains two photons O and X that currents erase each other, (b) an O-X-(O, X) oscillator in which in the static part (stat1) both photons O and X contain positive action and in the (dyn) part in both photons O and X contain negative action; the (stat1) and (dyn) contain the same amounts of action, and (c) an O-X-(O, X) oscillator in which in (stat1) in both photons O and X the action is negative and in (dyn) positive. While (a) the mass oscillators forms and in pure form presumably form the dark matter, (b) forms the negative charges and (c) the positive charges. Based on the ideas of the PIT, the three variants are formed simultaneously during a phase after the Big Bang [4] .
9. Comparison with the Higgs Fields
The formalism of the PIT is derived from the scalar Lagrange density, from the energy-momentum tensor of the Maxwell fields and from the associated four-dimensional commutators of the communication relations. Unlike quantum mechanics, where an elementary object is described by a wave function, a distinction is made between the fields of the object’s core and its photon cloud. The core is expressed by the scalar fields and the photon cloud by the Maxwell fields.
The Lagrange density of the Higgs fields is represented by the expression
[12] , where
and
are positive quantities and
has the dimension of a mass. The first two terms correspond to the Klein-Gordon equation, where the square of the mass has a negative sign and corresponds to an imaginary mass. The square part in the representation of L is assumed to be the interaction term between
and
with the interaction constant
.
We want to compare the description of the Higgs fields by the Lagrange density with the functioning of a mass oscillator. To do this, we identify the Higgs fields
and
with the PIT representation of an object consisting of a mass oscillator and its photon cloud. In our formalism, each field
and
includes an O or X photon, i.e., each O or X photon is correlated to an O-X (O, X) oscillator. O and X are conjugated complex and have opposite signs of action. This also applies to the products
and
. In the PIT, this is due to the representation of the scalar oscillators and the Maxwell fields on the Fourier space. Since each product
and
is associated with an O or X photon, the PIT describes in the product
of the Higgs field the interaction of the two photons O and X in an interaction between two O-X gravitons under formation of a delta of action. This interaction leads to the formation of the deltas of information and to the change of the rest frame.
The representation of the PIT is thus compatible with the representation of the Higgs mechanism in the Lagrange density. The complex mass results from its representation on the Fourier space; in space-time, the mass remains real. The two fields
and
are always linked to each other, because they are connected to each other via O and X with the formation of the O-X graviton and with the O-X gravitons of the photon cloud. This is comparable to the different masses of the heavy Higgs boson and the light Goldstone boson of quantum mechanics. Both contain mass because they contain the action of gravitons.
However, the PIT leads to a different interpretation of the physical process. While in the Higgs mechanism the mass is hindered by the Higgs field in its movement (see party representation of Alice and Bob), in the PIT the interaction with an increasing gravitational field causes an acceleration of the mass; with a homogeneous gravitational field, the content of the action in the object and its photon cloud and thus also the rest frame is preserved.
10. Summary and Discussion
Within the framework of the Theory of Physical Information (PIT), a model of a mass memory was discussed. Starting from the properties of a generator of action, which is formed by a μ = 0 oscillator in a three-dimensional structure by a four-dimensional Maxwell field and a scalar oscillator, whose mass is described in field-field correlations, a model of a mass oscillator was formed, which describes within the framework of the PIT’s ideas the properties of the mass in interaction with the gravitons. Under the assumption that one can describe the oscillation behaviour of masses under the conditions of the Hamilton principle with the help of the generation of action in a μ = 0 oscillator, described by the four-dimensional vector potential, the following statements are obtained:
The mass of a scalar oscillator in the present model consists of an oscillator, formed of two parts (stat1) and (dyn). The (stat1) part has the properties of gravitons, in that the two photons O and X have the same action with different signs, and the “dynamic” oscillator part (dyn), which also consists of two “dynamic” photons O and X with different signs of action of the same amount and the amount of virtual action is equal in the static part and dynamic part of the mass-oscillator. Both oscillator parts (stat1) and (dyn) overlap so that their direction of motion is the opposite. It is shown that their oscillation occurs differently than in the photons of static Maxwell fields and in photons of light and therefore the associated scalar field-field correlations form a stable state.
The mass oscillator contains a non-relativistic limit (NRL) that separates the range of non-relativistic acceleration from relativistic acceleration. As a result, in the mass oscillators there is a non-relativistic range below the non-relativistic limit (NRL), in which the non-relativistic behaviour is described, and a range above the NRL, which contains the relativistic behaviour. Above the NRL, in addition to the non-relativistic rest mass, mass is formed by absorption of action, which is detectable by increasing the action of gravitons in the photon cloud. The action accumulated above NRL is only temporarily stored and is emitted again when the relative speed is reduced below the NRL.
The absorption/emission of action leads to a change in the rest frame: if action is added in the memory of the mass, then the object is accelerated, the proportion of the action in the mass oscillator is reduced, then the relative velocity becomes lower. The virtual action is stored in the mass oscillator; the mass is stored virtual action. Under the conditions of the Hamilton principle in one oscillator-part the changes in the action (four-dimensional delta) are added up, with the addition of action that is gravitons in the photon cloud, in the other oscillator part of the oscillators the action is added in the mass oscillator. In a rest frame the action in the mass-oscillator is same, as in the photon cloud.
The inertia of the mass is caused by the stored action in the mass oscillators, because with the addition of action in the form of the four-dimensional deltas, contrary to the conditions of the Hamilton principle (minimization of action), a change in the currents in the scalar oscillators must be affected by supplying action. In the PIT, therefore, in contrast to the current discussion in physics, there is another mechanism of changing the rest frame; this is done by changing the virtual action in the scalar oscillators.
The scalar O-X (O, X)-oscillator in the form in which it is discussed in this report can also be regarded as the basis of harmonic structures that exist only in a vacuum, because it oscillates only by interacting with the gravitons of the vacuum, i.e., only by exchanging virtual action. The masses have a fixed value of the action in the O-X (O, X) oscillator, which does not have to be the case for similar structures in the vacuum. The mass oscillators were probably formed at a certain density of the activated virtual action after the Big Bang and are probably bound to a certain number of such oscillators in a fixed compound. However, in a vacuum, “mass oscillators” can also form, which contain a lower action. In particular, one can refer here to the oscillation of neutrinos, which are practically undetectable in vacuum and probably consist of some of the oscillators of the form (1).
Unlike quantum mechanics, where the Higgs field prevents elementary objects from moving at the speed of light, the PIT shows that it is the inertia of the mass of the elementary objects themselves that prevents the speed of light of the objects. The specific mass of objects is formed by the number of mass oscillators, with each mass oscillator having the same action. If the action of the mass oscillators (in thought) is reduced, the relative speed increases under otherwise identical conditions; with minimal action in the mass oscillators an object can reach almost the speed of light.