Ionic Liquid Efficiency on Wood Dissolution and Polysaccharide Identification ()
1. Introduction
Secondary cell wall polysaccharide is composed of 3 major components: cellulose, lignin and hemicelluloses to which is added extractives, pectin and proteins. Biomass composition and organization are a prerequisite for industrial valorization. Usual polysaccharide extractive methods are based on chemical, physical or enzymatic treatment or by a combination of several of them [1]. To overcome long and complex methods, we previously developed ionic liquid/ELISA process [2]. Ionic liquids (ILs) have generally been defined as substances entirely composed of ions having a melting temperature below 100˚C [3]. ILs are salts composed of anions and cations that can be easily modified leading to a number of potential cation/anion combinations permitting to estimate the number of possible different IL to be equal to 1012 [4]. ILs are known for their high thermal stability, low vapor pressure and good ability to dissolve polar, non-polar, organic or inorganic compounds [5] [6] giving us a wide range of applications [6] [7] [8].
In this study we compare the effect of several ILs on the wood dissolution process by the use of a set of plant cell wall antibodies. We synthesized 6 ILs to compare, for the first time, their efficiency to solubilize Douglas-fir sapwood. ILs synthetized are the following: 1-ethyl-3-methylimidazolium bromide ([Emim]Br), 1-ethyl-3-methylimidazolium acetate ([Emim]Ac) 1-allyl-3-methylimidazolium bromide ([Allmim]Br), 1-allyl-3-methylimidazolium chloride ([Allmim]Cl), butyl-1.8-diazabicyclo[5.4.0]undec-7-enebromide ([DBUC4]Br) and butyl-1.8-diazabicyclo[5.4.0]undec-7-ene chloride ([DBUC4]Cl). DBU based ILs were chosen because of the good ability of [DBUC4]Cl to dissolve lignin [9] and imidazolium ILs their good ability to dissolve wood [10] [11] [12]. To characterize IL compatibility to ELISA immune-essay, we first determined the sensitivity of 14 monoclonal antibodies (mAbs) using commercial polysaccharides dissolved in the 6 ILs. Each IL-wood solubilized solution was then analyzed with ELISA (Enzyme Linked Immuno Sorbent Assay) technique thanks to the previous mAbs to choose an IL for our application having a good ability to dissolve wood without degrading or modifying so much cell wall polymers.
2. Materials and Methods
2.1. Products and Material
1-methylimidazole (99%), bromoethane (98%), potassium acetate (99%), 1.8-diazabicyclo[5.4.0]undec-7-ene (99%), bromobutane (98%), allyl chloride (98%) were purchased from Alpha Aesar and allyl bromide (99%) stabilized and chlorobutane (99.5%) were purchased from Fischer Scientific. Ethyl acetate (99%) was purchased from Carlo Erba, dichloromethane (99.9%) from SDS, absolute ethanol from VWR and DMSO d6 with 0.03% tetramethylsylane (Me4Si, 99.8%D) from Eurisotop.
1H and 13C NMR spectra were recorded at 400.13 MHz and 100.62 MHz with a Bruker DPX-400 spectrometer using DMSO(d6) as solvent at room temperature. The chemical shifts (δ) are expressed in ppm with Me4Si as the internal standard (δ0).J values are given in Hz.
2.2. Ionic Liquid Synthesis
2.2.1. [Emim]Br
A mixture of 1-methylimidazole (41.06 g, 0.5 mol) and bromoethane (65.38 g, 0.6 mol) was stirred at 35˚C for 20 h. The product was washed with ethyl acetate (2 × 100 ml) and dried in a vacuum. Yield: 96% (91.72 g).
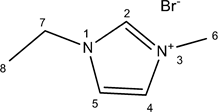
1H NMR (DMSO d6): δ 9.44 (1H, s, H-2), 7.92 (1H, t, H-5, J5,4 = 1.7), 7.81 (1H, t, J4,5 = 1.7), 4.22 (2H, q, J7,8 = 7.3), 3.87 (3H, s), 1.38 (3H, t, J8,7 = 7.3). 13C NMR (DMSO d6): δ 136.24 (C-2), 123.46 (C-4 or C-5), 121.92 (C-4 or C-5), 44.07 (C-7), 35.73 (C-6), 15.18 (C-8).
2.2.2. [Emim]Ac
[Emim]Ac was synthesized through the ion exchange reaction. Dried potassium acetate (37.75 g, 0.385 mol) was completely dissolved in 350 ml of ethanol before adding slowly [Emim]Br (70 g, 0.385 mol). The reaction is carried out at 40˚C at 5 h. The solution is then put at −20˚C at 1 h before being filtered to remove the potassium bromide precipitate. The ethanol was evaporated and the product was then placed at −20˚C for overnight and filtered again. The resulting pale yellow viscous liquid was obtained in 84% yield (52.1 g).
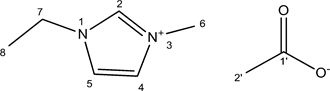
1H NMR (DMSO d6): δ 9.44 (1H, s, H-2), 7.92 (1H, t, H-5, J5,4 = 1.7), 7.81 (1H, t, J4,5 = 1.7), 4.22 (2H, q, J7,8 = 7.3), 3.87 (3H, s), 1.38 (3H, t, J8,7 = 7.3). 13C NMR (DMSO d6): δ 136.24 (C-2), 123.46 (C-4 or C-5), 121.92 (C-4 or C-5), 44.07 (C-7), 35.73 (C-6), 15.18 (C-8).
2.2.3. [Amim]Cl
Allyl chloride (11.65 g, 0.152 ml) was slowly added to 10 g (0.122 mol) of 1-methylimidazole (molar ratio 1.25:1) in a round-bottomed flask with a reflux condenser in an ice bath. After 12 h at 55˚C, unreacted chemical reagents were removed by evaporation to obtain a yellow viscous liquid in 97% yield (16.94 g).
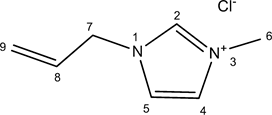
1H NMR (DMSO d6): δ 9.45 (1H, s, H-2), 7.83 (1H, t, H-5, J5,4 = 1.8), 7.81 (1H, t, H-4, J4,5 = 1.8), 6.06 (1H, ddt, H-8, J8,9B=16.9; J8,9A = 10.3; J8,7 = 6.0), 5.35 (1H, dq, H-9A, J9A,8 = 10.3; J9A,9B = 1.3), 5.30 (1H, dq, H-9B, J9B,8 = 16.9; J9B,9A = 1.3), 4.90 (2H, dt, H-7, J7,8 = 6.0), 3.90 (3H, s, H-6).
2.2.4. [Amim]Br
Allyl bromide (13.84 g, 0.114 mol) was slowly added to 7.5 g (0.092 mol) of 1-methylimidazole (molar ratio 1.25:1) in a round-bottomed flask with a reflux condenser in ice bath (the reaction is very exothermic). After 48 h at 22˚C, unreacted chemical reagents were removed using a rotary evaporator at 80˚C at 1 h. The resulting brown viscous liquid was obtained in 96% yield (21.06 g).
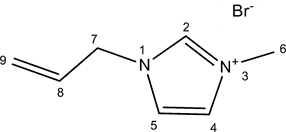
1H NMR (DMSO d6): δ 9.24 (1H, s, H-2), 7.79 (1H, t, H-5, J5,4 = 1.8), 7.76 (1H, t, H-4, J4,5 = 1.8), 6.06 (1H, ddt, H-8, J8,9B = 17.0; J8,9A = 10.3; J8,7 = 6.0), 5.36 (1H, dq, H-9A, J9A,8 = 10.3; J9A,9B = 1.3), 5.31 (1H, dq, H-9B, J9B,8 = 17.0; J9B,9A = 1.3), 4.89 (2H, dt, H-7, J7,8 = 6.0), 3.89 (3H, s, H-6).
2.2.5. [DBUC4]Cl
A mixture of DBU (40.8 g, 0.268 ml) and chlorobutane (29.77 g, 0.32 mol) was stirred at 70˚C for 72 h and then 20 h at 90˚C. The resulting orange gum was washed with dichloromethane (2 × 100 ml) and dried in a vacuum. Yield: 96% (63.07 g).

1H NMR (DMSO d6): δ 3.62 (2H, m, H-2), 3.50 (2H, t, H-12, J12,13 = 7.5), 3.47 (2H, t, 9-H, J9,10 = 5.7), 3.44 (2H, t, H-11, J11,10 = 5.7), 2.86 (2H, m, H-6H), 1.96 (2H, qn, H-10, J = 5.7), 1.66 (2H, m, H-4), 1.62 (4H, m, H-3 and H-5), 1.52 (2H, qn, H-13, J = 7.5), 1.30 (2H, sx, H-14, J = 7.5), 0.90 (3H, t, H-15, J15,14 = 7.5). 13C NMR (DMSO d6): δ 165.81 (C-7), 53.84 (C-2), 52.79 (C-12), 48.41 (C-11), 46.50 (C-9), 30.23 (C-13), 27.69 (C-4), 27.01 (C-6), 25.37 (C-3), 22.71 (C-5), 19.50 (C-10), 19.02 (C-14), 13.64 (C-15).
2.2.6. [DBUC4]Br
DBU (8.31 g, 0.055 mol) was introduced in a round flask placed in an ice bath. 1.2 equivalent of bromobutane (0.066 mol, 8.98 g) was slowly added under stirring. The flask was then fitted with a reflux condenser and put in an oil bath. The oil bath temperature was slowly increased until 80˚C at 2 h and 90˚C at 6 h. Unreacted chemical reagents were removed by evaporation at 80˚C at 1 h to give a brown solid in 96% yield (15.11 g).
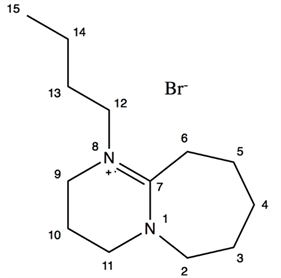
1H NMR (DMSO d6): δ 3.63 (2H, m, H-2), 3.50 (2H, t, H-12, J12,13 = 7.4), 3.48 (2H, t, H-9, J9,10 = 5.8), 3.45 (2H, t, H-11, J11,10 = 5.8), 2.86 (2H, m, H-6), 1.97 (2H, qn, H-10, J = 5.8), 1.68 (2H, m, H-4), 1.63 (4H, m, H-3 and H-5), 1.52 (2H, qn, H-13, J = 7.4), 1.30 (2H, sx, H-14, J = 7.4), 0.90 (3H, t, H-15, J15,14 = 7.4).
2.3. Wood Sampling and Dissolution
Wood samples (1 - 2 cm thick disks) were collected at 1.3 m from the trunk of a 20-year-old Douglas-fir tree (Pseudotsugamenziesii) in October at Gimel, France (45˚18'N, 1˚50'E). Once the bark was removed, the softwood part of the disks was grounded into a fine powder (<250 μm) with a Dangoumeau steel ball mill. Wood powder samples were then homogenized and stored in a dry atmosphere until analysis.
Douglas-fir softwood was dissolved at 10 mg per 5.23 mmol in each IL previously synthetized ([Emim]Ac, [Emim]Br, [Amim]Br, [Amim]Cl, [DBUC4]Br and [DBUC4]Cl). Wood dissolution has been performed at 80˚C or 100˚C until complete solubilization of wood particles. Dissolution advancement was followed by optical microscopy.
2.4. Polysaccharide Identification
Before The polysaccharide identification is made thanks to an ELISA test with a set of 14 monoclonal antibodies (mAbs) (Table 1).
Commercial polysaccharides, used as controls for ELISA tests, were dissolved in ionic liquid in the 6 pure ILs (10 mg∙mL−1) thanks to a heating treatment at 80˚C at 3 h. The following polysaccharides was used: guar from Cyamopsis
![]()
Table 1. Monoclonal antibodies (mAbs) characteristics.
tetragonoloba (Sigma-Aldrich), ß-(1-4)-mannopentaoses (Megazyme), xylans from birchwood (Sigma-Aldrich), xyloglucans from tamarin seeds (Megazyme), pectin from citrus (Sigma-Aldrich), arabic gum (Sigma-Aldrich), Karaya gum (Sigma-Aldrich), arabinans from sugar beet (Megazyme).
All dilutions of commercial or Douglas-fir polysaccharides were done in deionized water to decrease the viscosity of the IL-polysaccharide solution and facilitate an accuracy sampling. Diluted polysaccharides-IL or wood-IL solutions were applied to 96-well plates (Nunc, ref 269620 ThermoFischer) over a broad concentration range (0.5 ng to 5 µg for polysaccharides or 0.05 to 100 µg for wood solutions). Control wells were coated with deionized water with IL at the same concentration as in other polysaccharides-IL solutions wells. The liquid was then evaporated overnight at 37˚C. After a washing step with 300 µL Tris-buffer saline (TBS) (Tris-HCl 50 mM, NaCl 150 mM, pH 7.6), the plates were blocked with 300 µL of 1% (w/v) nonfat dry milk in TBS for 1 h. After having removed the blocking solution by manual turning, 50 µL of primary mAb (1:100 in TBS) were added to each well and incubated for 4 h at room temperature. The supernatant was removed and wells were washed three times with 300 µL of 0.1% (w/v) nonfat milk in TBS (wash buffer). Then, 50 µL of diluted (1:5000 in wash buffer) peroxidase-conjugated goat anti-mouse IgG or goat anti-rat IgG antibodies (Sigma-Aldrich), depending on the primary antibody used, were added to each well and incubated for 1 h. Wells were washed five times with 300 µL of wash buffers. 50 µL of 1-Step Ultra TMB (3.3’,5,5’-Tetramethylbenzidine) ELISA Substrate Solution (Life Technologies) were added to each well. The reaction was stopped by adding 50 µL of 0.5 N sulfuric acid. The OD of each was read well as the difference in A450 and A655 using a microplate reader (BMG-Labtech, FLUOstar Omega).
3. Results
3.1. Abbreviations and Acronyms
Six ILs, [Emim]Ac, [Emim]Br, [Amim]Br, [Amim]Cl, [DBUC4]Br and [DBUC4]Cl were synthesized to compare their efficiency for dissolving Douglas-fir sapwood as described in material and methods. These ILs have been chosen because of their good ability to solubilize either cellulose, lignin or even wood. Firstly, [Emim]Br was synthesized as a precursor for the synthesis of [Emim]Ac. [Emim]Ac was synthesized from [Emim]Br through the ion exchange reaction according to [23] adapted by [24] plus an increase of the reaction time (40 h instead of 5 h).
Two other imidazolium-based ILs were synthesized, [Allmim]Cl and [Allmim]Br. The first one was synthesized according to [25] and the second one, [Allmim]Br, result of the reaction of allyl bromide and 1-methylimidazole. These two ILs were obtained with a 100% purity, and in 97% and 96% yields for [Allmim]Cl and [Allmim]Br respectively.
DBU-based ILs, [DBUC4]Cl and [DBUC4]Br were synthesized from 1 equivalent of DBU and 1.2 equivalents of chlorobutane or bromobutane. They were obtained pure, in 96% yield.
3.2. Full Wood Dissolution
Douglas-fir softwood dissolution has been performed at two heating temperatures: 80˚C and 100˚C (Figure 1).
The wood particles dissolution was followed by optical microscopy as shown in Figure 1 for to [Emim]Br. Identical results were obtained with each of the 6 ionic liquids (Table 2).
Our dissolution results show that the time to obtain a complete dissolution varies greatly depending on the IL and the treatment chosen. Usually for the 5 ILs, the time to dissolve the wood particles decreases with the increase of temperature from 80˚C to 100˚C. At a temperature dissolution of 80˚C, the ILs the most efficient are [Emim]Ac and [Allmim]Br for which 54 h is enough to completely dissolve wood. At this temperature, the least efficient are [Emim]Br and [DBUC4]Br. At 100˚C, [Allmim]Br solubilizes Douglas-fir wood in only 14 h, whereas this time is 2-fold higher for [Emim]Ac and at least 6-fold higher for the other ILs.
3.3. Commercial Polysaccharide Identification
The ELISA tests were carried out on a panel of pure commercial polymers previously dissolved in the 6 ionic liquids as described in material and methods. Labeling was observed for each of the commercial polymers, independently of
![]()
Figure 1. Dissolution kinetics of Douglas-fir sapwood particles in [Emim]Br at 80˚C (a) and 100˚C (b). Scale bar: 150 μm.
![]()
Table 2. Times to obtain a complete dissolution of Douglas-fir sapwood particles in the different ILs depending on the treatment.
the ionic liquid used. The 6 ILs tested do not interfere with the polymer recognition by antibodies and the labeling is proportional to the quantity of polymer deposited on the ELISA plate. For commercial pure polymers, the regression lines have regression coefficients greater than 0.95. The labeling is thus proportional to the polymer amount deposited, whatever the IL. However, variations in the sensitivity of the method (minimum amount of polymer detected) have been identified. Moreover, the pectin solubilized in [Emim]Ac induced some chemical modifications, such as caramelization of the solution, suggesting strong pectin polysaccharide degradation or chemical reactions between the polymers and the IL in our experimental conditions.
3.4. Ionic Liquid Efficiency
This technique has been then applied to Douglas-fir wood samples. The results are presented for the same antibody in the form of relative percentage of labeling (DO) for each of the ILs (Figure 2). For the same antibody, labeling intensity differs depending on the IL used for dissolving the wood. In the case of [DBUC4]Br with a dissolution temperature of 80˚C (Figure 2), low labeling is observed with all antibodies. Some pectin and XG labeling disappear with the increase of temperature to 100˚C. At 80˚C, the labeling is generally greater with [DBUC4]Cl, IL for which the pectin labeling (HGs and RGI) are among the strongest of the 6 ILs studied. Moreover, the [DBUC4]Cl and the [DBUC4]Br solution show a high viscosity level after wood dissolution. Wood dissolved in the
![]()
Figure 2. Relative percentages of labeling for each antibody after wood dissolution in each of the 6 ILs at 80˚C (a) or 100˚C (b).
[Amim]Cl has a low labeling intensity for pectin (HGs and RGI) and xylans. Labeling intensities are also weak or nonexistent in the case of [Emim]Ac whatever the temperature. Comparing the results for the [Emim]Br and [Amim]Br at 80˚C, a more important labeling is observed for pectin, AGP and galactan epitopes for the wood dissolved in [Amim]Br, while the labeling is higher in the case of [Emim]Br for arabinan, XG and mannan epitopes. At a dissolution temperature of 100˚C, some pectin labeling disappears in the case of [Emim]Br. Only wood dissolved at 100˚C in [Amim]Br has a label with CCRC-M14, LM20 and JIM7 antibodies. However, in this LI, the labeling is lower than in [Emim]Br for xylan, mannan, arabinan and XG epitopes. These two ILs have interesting characteristics for the solubilization and detection of wood polymers. A dissolution temperature of 80˚C is preferred because of a lack of labeling for some polymers after dissolution at 100˚C. This fact involves a possible degradation of these polymers or modifications of the epitopes recognized by the antibodies after a dissolution at 100˚C. This degradation/modification is also possible at 80˚C.
However, to the extent that all the wood samples will be treated in the same way (same temperature and dissolution time), this degradation/modification should be in the same proportion for all samples enabling the comparison of their biochemical composition using IL/ELISA method.
3.5. Wood Polysaccharide Identification
Six days at 80˚C in [Emim]Br allow a total dissolution of the biomass previously reduced to powder in the Dangoumeau steel ball mill. In contrast, only 3 days should be necessary after liquid nitrogen grinding. New tests were therefore conducted with the [Emim]Br from earlywood and latewood powder by combining the treatment at 80˚C and 100˚C in order to see if there was an improvement in the sensitivity of the method (only some of the 14 antibodies were chosen, one per polymer family). The following treatments were therefore compared: 3 days of dissolution at 80˚C, 4 days of dissolution at 80˚C, 3 days of dissolution at 80˚C followed by 2 h at 100˚C and 4 days of dissolution at 80˚C followed by 2 h at 100˚C (Figure 3(a) and Figure 3(b)).
Wood dissolution and ELISA analyses were done in triplicate. The standard deviation of repeated measurements is rather low (Figure 3(a)), even if some standard deviations are higher for some samples (for example LM11, 3 days at 80˚C) or for one antibody (particularly LM25). Whatever, the method stays reproducible for all the antibodies. Regarding the results for the sapwood earlywood (Figure 3), labeling is comparable regardless of the dissolution treatment for CCRC-M14, LM25, LM19, LM11 and LM21 antibodies. The LM5 labeling is more intense for the 3-day treatment at 80˚C in the case of the sapwood earlywood (Figure 3(a)) and the heartwood latewood (Figure 3(b)) than the other treatments. For the sapwood earlywood, a labeling difference is also observed for LM21 and LM11 for which labeling are more intense after 4 days at 80˚C followed
![]()
Figure 3. Labeling intensity (OD) with some antibodies function of time and temperature of the dissolution of Douglas fir ((a) sapwood earlywood ring; (b) heartwood latewood ring). Quantities of wood dissolved: 10 µg for CCRC-M14 and LM19, LM25 and 5 µg for LM5 0.5 µg for LM11 and LM21 0.2 µg. Dissolution and ELISA were done in triplicate.
or not by 2 hours at 100˚C. However, the labeling differences between treatments are low, which shows that these differences in dissolution time and temperature only slightly impact the dissolution. process or polymer degradation in this IL.
3.6. 16-Year-Old Polysaccharide Monitoring
The composition of Douglas-fir was analyzed in triplicate after separating earlywood from latewood of each of the rings (Figure 4). Concerning the HGs (CCRC-M38, LM19 and JIM7 antibodies, Figure 4), the labeling trends remain the same. This test also made it possible to see differences in terms of the proportion
![]()
Figure 4. Labeling intensity (DO) for homogalacturonan (CCRC-M38, LM19, JIM7), rhamnogalacturonans (CCRC-M14, CCRC-M35), xyloglucans (LM15, LM25, CCRC-M1), AGPs (LM2), galactans (LM5) and arabinans (LM6) and xylans (LM10, LM11) for earlywood (i) and latewood (f) of each of the rings of a slice of Douglas-fir from the external sapwood (ring 1) to the pith (16). Dissolution in triplicate in [Emim]Br 4 days at 80˚C.
of HGs according to the type of wood. Indeed, higher proportions are observed in earlywood compared to latewood. No labeling could be visualized for the LM20 antibody. The labeling trends observed for the RG I (Figure 4) are almost identical for the three repeats in the case of the CCRC-M14 antibody. Variations appear for one of the repeats in the case of CCRC-M35, but the trends remain similar. Higher proportions of RG I are noted in early wood. Regarding xyloglucans, the technique is reproducible for the LM25 antibody.
On the other hand, some labeling variations are observed between the repetitions in the case of fucosylated xyloglucans (CCRC-M1) and the technique is not reproducible with the LM15 antibody (Figure 4). This can be explained by a sensitivity limit of the method due to low proportions of xyloglucan epitopes recognized by CCRC-M1 and LM15 in the wood (the intensities of DO do not exceed 0.3 for a deposited quantity of 20 μg of dissolved wood) or by a lack of homogeneity of the solubilization in the IL of the XGs with XLFG, XFFG, XXFG and XXXG pattern or even by a lack of affinity of these polymers for the polycarbonate of the ELISA plates. The results obtained with the LM25 antibody show that the proportion of xyloglucans is greater in early wood than in latewood. In the case of AGPs (LM2, Figure 4), variations are observed between the labeling of the three repetitions, the technique is therefore not reproducible for these polymers. The same reasons as those previously invoked for the XGs can explain this lack of reproducibility. Even if labeling variations are observed for some samples for galactans and arabinans, overall, the labeling follows similar trends (LM5 and LM6, Figure 4). Regarding galactans, higher proportions are observed in the latewood of the sapwood, whereas for the heartwood, this trend is reversed. In the case of arabinans, the marking is much more intense in the early wood of the sapwood than in its latewood, on the other hand the trends are less clear-cut in the heartwood. The labeling intensities for the xylans (LM10 and LM11, Figure 4) show variations between the repetitions for the same ring. These variations are all the more marked in the case of LM11. A lack of homogeneity in the dissolution of the wood by the LI, in particular in the solubilization of this polymer, can therefore occur. Be that as it may, labeling trends remain visible with the LM10 antibody. Moreover, these results would tend to show that the xylans are in greater proportion in the early wood, in particular at the level of the sapwood (the trends are less clear for the rings most in the center of the heartwood). In the case of mannans (LM21 and LM22 antibodies, Figure 4), the first repetition gives different results for many tree rings compared to the data collected for repetitions 2 and 3, in particular for the LM22 antibody. On the other hand, the trends in terms of labeling intensity observed for repetitions 2 and 3 are similar, despite some differences in values. The solubilization of mannans in [Emim]Br therefore does not always appear homogeneous. The labeling intensities with LM21 show that the mannans are in greater proportion in the latewood than in the early wood.
4. Discussion
The ILs cation is generally synthesized by alkylation of an atom bearing a lone pair (nitrogen, phosphorus or sulphur). Although the heart of the cation is often the same, diversity lies in the length of the alkyl chains [26]. Thanks to this method, we synthesized five pure ILs [Emim]Br, [Amim]Br, [Amim]Cl, [DBUC4]Br and [DBUC4]Cl. Anion modification occurs by exchange with an alkali metal ion or an alkali salt. This is how we have synthesized [Emim]Ac, by exchange between the anion bromide of [Emim]Br and acetate anions of potassium acetate following as described in material and methods. To slightly improve the purity of the IL, we increased the time reaction (24 h instead of 5 h) and managed to obtain [Emim]Ac pure only at 89% (the purity was 85% for a reaction time of 5 h).
We have tested the effectiveness to dissolve the Douglas fir wood in 6 ILs with imidazolium cation ([Emim]Br, [Emim]Ac, [Amim]Cl and [Amim]Br) or DBU cation ([DBUC4]Br and [DBUC4]Cl). At 80˚C, we observed that the most efficient ILs was [Emim]Ac and [Amim]Cl (56 h), followed by [Amim]Br and [DBUC4]Cl (96 h), the least effective being [Emim]Br and ([DBUC4]Br (6 days). By increasing the dissolution temperature to 100˚C, the time necessary to obtain complete Douglas-fir wood dissolution decreases whatever the IL, following the same hierarchy of efficiency as measured at 80˚C. As we can see, the dissolution temperature affects the process and the dissolution rate. The viscosity decreases with increasing temperature facilitating the breaking of intra and interchain hydrogen bonds [27] leading to an increase in the rate of dissolution of woody biomass as suggested [28].
Comparing the efficiency of the cation (for [Amim]Br, [Emim]Br and [DBUC4]Br), we conclude from our study that the cation [Amim] is more efficient to dissolve Douglas-fir wood that [Emim] and [DBUC4], which have similar efficiency. The imidazolium cation with its electron rich aromatic π system may interact with cellulose hydroxyl oxygen atoms, preventing cross linking of the cellulose molecules [25] [29] [30]. Higher efficiency of [DBU] and [Emim] cations, probably due to an electron displacement on the imidazole as in side chain [28]. Acetate and chloride were more efficient than the bromide whatever the cation. These parameters are determined by measuring the UV-Vis spectra of dyes when dissolved in a solvent of interest. In this system, β is the parameter describing the hydrogen-bond basicity, α is a measure of the solvents’ hydrogen-bond acidity and π* of its interactions through bipolarity and polarizability [31]. In ionic liquids, β is primarily influenced by the anion [32]. For cellulose solubility, it was shown that ionic liquids that dissolve cellulose are characterized by high values for parameters describing solvent hydrogen-bond basicity [33], and that chloride anion has a higher hydrogen-bond basicity (0.97) than bromide anions (0.90) [34].
With [Emim] Ac we obtained a complete dissolution of Douglas-fir wood particles in 54 h at 80˚C and 28 h at 100˚C. With a commercial solution of [Emim]Ac, [12] managed to completely dissolve red oak and pine wood powder (125 - 250 microns) after 25 h and 46 h respectively, at 110˚C. Sun et al. [12] suggested that wood composition could be correlated to cell wall destructuration efficiency. Although hardwood is generally denser than softwood, it generally contains less lignin [35]. In the wood, the majority of the lignin is linked to hemicelluloses and forms like a cement making an impenetrable structure [36]. The difference can also be explained by variations in interactions between lignin and polysaccharides, distribution of lignin or the unitary structure of this polymer (softwoods have lignin composed mainly of G units while hardwoods contain G and S units) [35]. Nevertheless, our results with the [Emim]Ac on Douglas fir wood are closer to those obtained for the Red Oak, although Douglas fir is a softwood and the IL used is not pure.
In terms of [Amim]Cl, it was shown that this IL could completely dissolve the pine wood powder (8% w/w; particle size: 0.1 - 2 mm) after 8 h at 80˚C [37]. At this temperature, 54 h were needed to dissolve finer particles (<250 µm) of Douglas-fir wood. This efficiency difference between these results can be explained because of the presence of water in our IL as demonstrated [11]. In our case, the wood was lyophilized but we have not sought to maintain the ILs before and during the dissolution process at their minimum water content, with the aim of simplifying, subsequently, the dissolution step of a large number of samples. Most hydrophilic ILs can absorb up to 10% of their water mass when they are exposed to the atmosphere at room temperature over 4 h [6] [27]. 3% to 5% of water block Miscanthus biomass dissolution in [Emim]Ac and [Emim]Cl [38]. Swatloski et al. [39] demonstrated that watered IL decrease cellulose solubility.
DBUC4 based ionic liquids were never used to solubilize wood, but [DBUC4]Cl was synthesized and used to dissolve lignin [9]. These authors determined the time of the dissolution of lignin in the [DBUC4]Cl, the [DBUC6]Cl and [DBUC8]Cl with lignin samples came from a precipitation with CO2 from a black liquor extract. At 105˚C, this lignin (20% (w/w)) is completely dissolved in the [DBUC4]Cl after 29 h. For a given percentage of lignin, it was shown that the dissolution time increases with the length of the alkyl chain of DBU.
4.1. Polymer Identification
200 monoclonal antibodies were able to detect a large amount of polysaccharide [40]. In this study, we focused on 14 antibodies able to react against hemicelluloses, pectin, and parietal proteins (AGPs) epitopes.
Solubilized polysaccharides have better accessibility of epitopes to antibodies [13]. However, the solvents used to solubilize or purify the cell wall polymers may lead to damage or changes in the chemical structure of epitopes recognized by antibodies [40]. We observed, after the dissolution of Douglas fir wood in various ILs at different temperatures, different labeling for the same antibody according to the IL.
Before analyzing by ELISA the Douglas fir wood dissolved in the various ILs, we realized commercial polymer ranges in order to observe the proportionality of the labeling depending on the amount of polymer deposited. We found that the 6 ILs used ([Emim]Br, [Emim]Ac, [Amim]Br, [Amim]Cl, [DBUC4]Br and [DBUC4]Cl) do not interfere with the polymer recognition by the antibodies and the labeling is proportional to the amount of polymer deposited on ELISA plates, despite some differences in the detection threshold based on ILs.
Wood samples completely dissolved in each of the ILs were then analyzed with the set of the 14 antibodies. In the presence of [DBUC4]Br, the labelling are weak whereas [DBUC4]Cl are the best, the [DBUC4]Cl and the [DBUC4]Br were not selected because their too high viscosity making the pipetting complicated. Low labeling was observed for pectin after wood dissolution in [Amim]Cl and [Emim]Ac at 80˚C and 100˚C. Wood solution dissolved in [Emim]Br and [Amim]Br at 80˚C present interesting labeling levels for all polymers. For higher temperature pectin labeling disappears in [Emim]Br. For xylans and mannans higher temperature is higher labelling is observed. This shows that, in the solutions obtained after wood dissolution at 80˚C, some mannan and xylan epitopes remain inaccessible to antibodies. Thus, a higher heating temperature allows a better dissociation of these hemicelluloses from other cell wall components. In softwood, (galacto)glucomannans exhibited similar orientation than cellulose microfibrils increasing linkeage [41] [42]. Moreover, (Arabino)glucuronoxylan and (glucurono)arabinoxylans are linked to lignin via ester bonds at the uronic acids of their side chains [43] [44]. Hence, higher wood dissolution temperatures in ILs would better separate mannans and xylans from cellulose and lignin.
In some case, high temperature treatment can lead to damage of certain polymers such as pectin or chemical changes of the epitopes recognized by antibodies. Microwave treatment can also lead to the same result. Walnut wood dissolves in [Emim]Ac, exhibited a decreased labeling for homogalacturonan (HGs) after a microwave treatment before conventional heating [2]. Sosha et al. [45] demonstrate that high temperatures (160˚C) degrease immunolabelling of HG (CCRC-M38, JIM5, JIM7 among others), rhamnogalacturonan I (RGI) and some arabinogalactan epitopes. However, after a pretreatment with the [Emim]Ac, galactomannan epitopes could be detected as xylan and xyloglucan.
Pine and oak cellulose solubilized [Emim]Ac, found minor degradation after 16 h at 110˚C [12]. The acetate of [Emim]Ac can also can modify polymers and hide epitopes. This has been shown for cellulose: after 20 min at 150˚C, the cellulose has an acetyl degree of substitution of 0.017 [46]. Yang et al., [47] shown partial degradation of hemicelluloses and lignin during bamboo solubilization in [Amim]Cl. The Douglas-fir wood solution dissolved in [Amim]Cl at 80˚C has one of the lowest xylan labeling. Pre-treatments with AFEX (ammonia fiber expansion) before sequential extraction induced increase of substituted or unsubstituted xylan epitopes are important, whereas the pectin epitopes (HGs and RGI) disappear [48].
Six days at 80˚C in [Emim]Br were necessary to completely dissolve the wood powder obtained after grinding with the Dangoumeau. In contrast, only 3 days in this IL were enough to completely dissolve sapwood or heartwood ground in liquid nitrogen in the ball-mill Retsch, due to a finer powder. By comparing immunostaining obtained after a dissolution of earlywood and latewood powder for 3 or 4 days at 80˚C, followed or not by 2 h at 100˚C, we found few differences with a limited number of antibodies (6). Increasing the processing time causes a decrease in the galactan epitope labeling and an increase for xylan and mannan epitope labeling. Labeling differences between treatments are, however, low, indicating that these differences in dissolution time and temperature impact very little solubilization and/or polymer degradation. Increasing dissolution time allows a better solubilization for mannans and xylans. Adding a step of 2 hours at 100˚C after a treatment at 80˚C has no impact on the immunolabeling result.
4.2. Conclusion
Different imidazolium or DBU based IL was synthesized and their efficiency to dissolve Douglas fir wood powder by heating at 80˚C and 100˚C was compared. [Amim]Cl and [Emim]Ac are the most efficient. However, these ILs lead to degradation or chemical modifications of wood polymers. The dissolution process at 80˚C in [Amim]Br, [Emim]Br and [DBUC4]Cl needs more time but polymers seem to be less modified. We showed, moreover, with [Emim]Br that the IL/ELISA method is reproductive to analyze plant cell wall composition after IL dissolution. Hence, this method was used to analyze Douglas fir wood composition from the outer sapwood to the inner heartwood, ring by ring, from wood cores, after a treatment of 4 days at 80˚C in [Emim]Br.