Distribution of Larger Benthic Foraminifera (LBF) in Selected Islands of Marine National Park, Port Blair, South Andaman, India ()
1. Introduction
Andaman and Nicobar group of Islands are located about 1500 km from the mainland consisting of 672 Islands with latitude 6˚ - 17˚N and longitude 93˚ - 94˚E. From this archipelago, 105 islands have been listed under Marine Protected Areas (MPAs) for their unique marine biodiversity. Further, salt water crocodile proliferation (man and animal conflict) is also one of the major concerns on these areas. Mahatma Gandhi Marine National Park (MGMNP) is situated at Wandoor, which belongs to the South Andaman District Administration, which is part of the Indian Union Territory (UT). MGMNP was established on 24th may 1983 under the wildlife Protection Act of 1972 to protect marine life such as the corals and nesting sea turtles prevalent in the area. The open creeks running through the park is the special attraction. It is 16 kms towards south of Port Blair, covering about 281.5 km2 area. It was placed under the protection of the Chief Conservator of Forest (Wildlife) of the forest department of the Andaman and Nicobar Islands. This Marine National Park comprises of 15 smaller group of islands with fringing type of coral reef and diverse form of associated flora and fauna. Type and composition of vegetation vary from island to island [1]. As these small group of islands fall under the MGMNP and not much study has conducted on the prevailing environmental condition of the coral reef, nor any monitoring study based on bio indicator organism. Globally, coastal zones are exposed to various anthropogenic activity likely, overfishing, pollution or tourism—recreational activity, that may pose thread to the prevailing environment [2]. So, a simplified monitoring mechanism essential to protect this environment is effected by the above factors.
Foraminifera constitute an inexpensive and easily abundant proxy for monitoring coastal environmental stressors, including elevated temperatures, acidification, and influx of pollutant. The symbiont-bearing benthic foraminifera are comparatively large, unicellular protist with test and lifestyle similar to that of corals, bearing zooxanthella [3] [4]. Experimental work carried out by Prazere et al., [5] showed Amphisteginalobifera from inner shelf able to acclimatize to wide nutrient level and larger temperature variation, but from mid or outer shelf of reef showed sensitive to any elevation of temperature and variation in nitrate. Dinoflagellate bearing Marginoporavertebralis is regarded as indicative species for “blue-water” condition, thus sensitive to nutrients than diatom bearing Calcarina species or Heterostegina species [6] [7]. Increased in concentration of carbon-di-oxide (CO2) up to 40% since pre-industrial period [8] likely result in decrease in pH (ocean acidification) affecting the calcifying organisms. Study by Vogel and Uthicke [9] found that decreased pH had no much effect on the growth rate of diatom bearing Amphisteginaradiata and Heterosteginadepressaand increased calcification was observed in dinoflagellate bearing Marginoporavertebralis and chlorophyta bearingAmphisorus hemprichii [10]. Later, study by Doo et al., [11] stated that decrease in calcification rate in porcelaneous, dinoflagellate-bearing foraminifera was noted with decreasing pH, while the hyaline diatom-bearing species showed no effect or increased calcification.
Further, study conducted by Talge and Hallock [12] and Schmidt et al., [13] showed that sensitivity of genus Amphisteginatowards bleaching showed light coloration with molting test without becoming completely white. Renema [7] generalized that diatom bearing hyaline LBF accommodate environmental changes than the dinoflagellate bearing porcelaneous LBFs. Hallock et al., [14] reported that response of coral reef on environmental changes is just a part of response to gradual changing condition to which LBFs are much sensitive than the reefs. Any changes in their substrate may affect their assemblage and alteration in benthic habitat structure [6] [7] [15] [16] [17]. With substrate change, larger impact may be seen in individual LBF species in response to ocean warming, ocean acidification and nitrification [2].
Hallock [18] already remarked the extensive presence of calcarinids in reef communities from Indo-Pacific regions. According to Hallock et al., [14], the distribution and abundance of the tests of symbiont-bearing benthic foraminifera can offer valuable clues to the health of a coral-reef ecosystem [19]. The well-known genus of symbiont-bearing foraminifera found worldwide on coral reefs and other shallow, tropical carbonate banks and its hardgrounds is Amphistegina [20] [21] [22]. Members of this genus are known to host diatom as their endosymbionts [4] [23] [24]. Any changes in local foraminiferal assemblages associated with coral reef environment may help in differentiating the deteriorating water quality from a temporary condition leading to an episodic mortality event [2] [25] in long term. Due to their abundance and species diversity, these microorganisms are reliable indicators of environmental disturbance and thus, reef foraminifera can be utilized as “bioindicators of coral-reef health” [26].
Therefore, the present study was carried out to enhance the knowledge on the diversity, abundance and depth distribution of larger benthic foraminifera (LBF) from six different islands in Mahatma Gandhi Marine National Park (MGMNP) and create a baseline data of the existing LBF in these prevailing environments.
2. Methodology
The study was undertaken to understand the LBF distribution and its environment from a protected area of Mahatma Gandhi Marine National Park (MGMNP), Wandoor, Port Blair, South Andaman (Figure 1), during the period of May-June, 2018, due to their very minimal anthropogenic impact on this region. Six islands with fringing coral reef environment were considered for the study. The fringing reef extended on the steep walls of submerged platform within 12 to 20 m depth ranges. The sediments were randomly collected by SCUBA divers on the sediments that extended up to 200 to 300 m width of the sea floor. Maximum two sediment samples were collected from each island, wherever thick sediment was deposited, otherwise only one sample was collected. This sediment was collected only one time during the study period. The collected sediments were brought to the laboratory and some amount of the sediment sample was spread in the tray
and live LBF were hand-picked. About 300 individuals of LBF species were collected, and studied. The collected LBF was analyzed under stereo binocular microscope and taxonomical identification was done using Loeblich and Tappan [27], and Milker and Schmieldi [28] foramininferal identification keys. For the investigation of species-environment relations, statistical method like Cluster analysis, Principal Component Analysis (PCA), and Multi-Dimensional Scaling (MDS) analysis were carried out and their results were interpreted. Table 1 represented the individual stations locations, depth and sediment texture.
3. Result and Discussion
Over all this study identified 22 LBF species from this environment (Table 2). However, their distribution varies with represent to the individual island environment. They are classified more than 5% in total percentage are presented in Table 3, and similarly, less than 5%, which are also available in all the studied stations are grouped in Table 4, for discussion. Table 5 represented by LBF and its symbionts available in varying depths. Plate 1 (Fig A to N) represented hyaline LBF and Plate 2 (A to H) represented the porcelaneous LBF.
Station 1—Snob Island (11˚34.112'N; 92˚34.631'E)
This island has a coastline cover of 2.18 km with an area coverage of about 17.65 Ha, having a length of 0.96 km and width of 0.26 km. It is one of the Island
![]()
Table 1. Study station with Latitude-Longitude, depth and sediment texture.
![]()
Table 2. Distribution of LBF species in the studied stations are represented by total percentage.
ND—Not Detected.
![]()
Table 3. LBF species observed more than 5% in all the stations.
ND—Not Detected.
![]()
Table 4. LBF species observed less than 5% in all the stations.
ND—Not Detected.
![]()
Table 5. LBF species and its associated endosymbiont from varying depth.
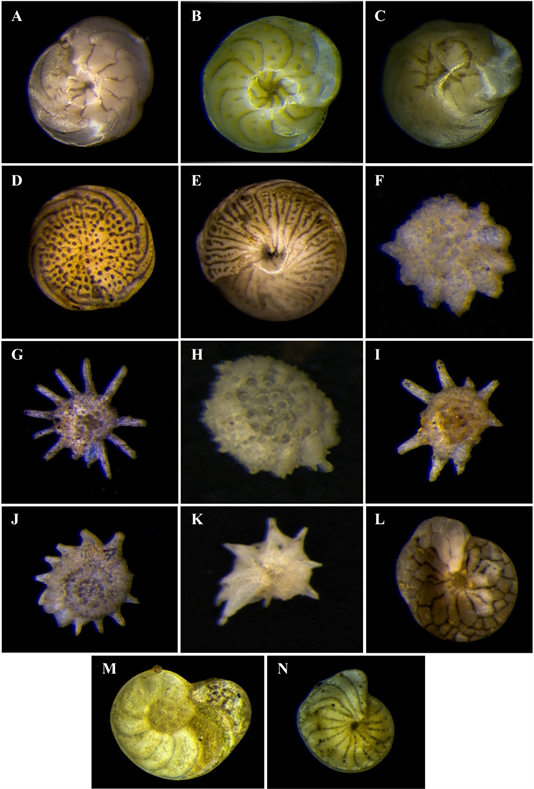
Plate 1. Hyaline LBF. Images of hyaline LBF from the study. (A) Amphisteginabicirculata Larsen, 1976 (B) Amphisteginalessonii d’Orbigny in Guerin-Meneville, 1832 (C) Amphisteginalobifera Larsen, 1976 (D)Amphistegina papillosa Said, 1949(E) Amphisteginaradiata (Fichtel & Moll, 1798) (F) Calcarina calcarinoides (Cheng & Zheng, 1978) (G) Calcarina defrancei d’Orbigny, 1826 (H) Calcarinahispida Brady, 1876 (I) Calcarina spengleri (Gmelin, 1791) (J) Neorotalia calcar (d’Orbigny in Deshayes, 1830) (K) Neorotaliagaimardi (d’Orbigny in Fornasini, 1908) (L) Heterosteginadepressa d’Orbigny, 1826 M. Operculinaammonoides (Gronovius, 1781) (N) Nummulitesvenosus, Fichtel & Moll, 1798.
included as part of Mahatma Gandhi National Park and known to have a good coral cover and biodiversity. Two samples were collected from this station, where both the samples showed seven dominant LBF species, respectively (Table 3). The LBF species are as follows: Calcarinadefrancei,C. spengleri,Neorotalia
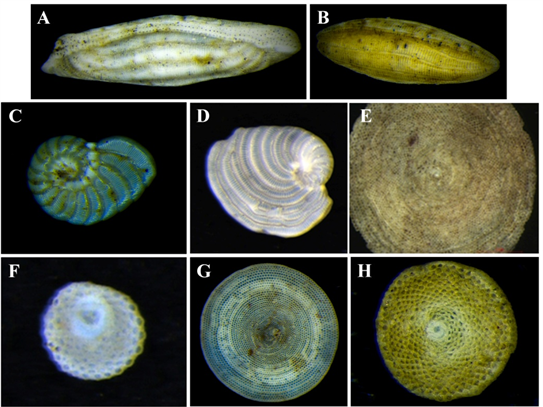
Plate 2. Hyaline LBF. Images of porcelaneous walled LBF from the study. (A) Alveolinella quoyi (d’Orbigny, 1826) (B) Borelis schlumbergeri (Reichel, 1937) (C) Peneroplis pertusus (Forsskal in Niebuhr, 1775) (D) Peneroplis planatus (Fichtel & Moll, 1798) (E) Amphisorus hemprichii Ehrenberg, 1839 (F) Marginopora vertebralis Quoy & Gaimard, 1830 (G) Parasorites orbitolitoides (Hofker, 1930) (H) Sorites orbiculus (Forsskal in Niebuhr, 1775).
calcar,Neorotalia gaimardi, Marginopora vertebralis and Sorites orbiculus. They found to be common in both the samples. Out of the dominant LBF species, Peneroplispertusus was observed exclusively only in the first sample, while Calcarinacalcarinoides has seen only in the second sample.
Station 2—Grub Island (11˚35.474'N; 92˚35.712'E)
This island is also a part of Mahatma Gandhi Marine National Park with total area of about 2.3 Ha and coastline cover of 0.7 km. It has a length of about 0.13 km and width of 0.25 km. It is home for spectacular coral reef environment. From the two samples, six dominant LBF species (Table 3), i.e., Calcarinacalcarinoides,Calcarina defrancei,Calcarina spengleri,Neorotalia calcar, Penoroplispertusus and Soritesorbiculus are present.
Station 3—Boat Island (11˚30.679'N; 92˚34.076'E)
This island comprises of 247.6 Ha landmass with a coastline cover of 7.71 km, length of 2.7 km and width of 1.3 km. Two samplings were carried out from this station, where the number of dominant LBF species was documented to be seven (Table 3). They are Calcarinacalcarinoides,Calcarina defrancei, C. spengleri, Neorotaliacalcar, N. gaimardi, Peneroplispertusus and Soritesorbiculus, whereas it has present only in the first sample.
Station 4—Hobday Island (11˚33.982'N; 92˚37.154'E)
This station has an area cover of 367.80 Ha with a coastline 10.50 km, length of 3.23 km width of 1.91 km. It has only one sample available with total of six dominant LBF species (Table 3). They are Calcarinacalcarinoides,C. defrancei,C. spengleri,Neorotalia calcar,Marginopora vertebralis and Soritesorbiculus.
Station 5—Belle Island (11˚33.854'N; 92˚33.906'E)
This island has a land area of about 6.7 Ha along with coastline cover of 1 km and length 0.38 km and width 0.21 km. Even this station had only one sample which showed dominance of seven LBF species, Calcarinacalcarinoides,C. defrancei,C. spengleri,Neorotalia calcar,Peneroplis pertusus,Marginopora vertebralis andSorites orbiculus (Table 3).
Station 6—Jolly Buoy (11˚30.663'N; 92˚37.022'E)
This island has an area of 18.80 Ha and a coastline cover of 2.50 km with a length 1.10 km and width 0.20 km, well known for its ecotourism. Two samples were collected and showed dominance of five LBF species (Table 3). The LBF species Neorotaliacalcar,Neorotalia gaimardi,Peneroplis pertusus,Marginopora vertebralis andSorites orbiculus are dominant in this environment.
Over all, compared all the study area, there are 22 species of LBF identified from these studied environments. Among these, the species Calcarinacalcarinoides,Calcarina defrancei,Calcarina spengleri,Neorotalia calcar,Peneroplis pertusus,Marginopora vertebralis andSorites orbiculus are present in considerable amount in all the study locations or except one location. These seven species extended almost same level of concentration in all these six studied island environments.
According to Renema [17], Calcarinaspecies are found in abundance in coral covered environment with adequate algal cover or algal turf, showing distinct habitat with reference to water quality, substrate type and bathymetry. Even, Hohenegger, [16] and Renema and Troelstra, [45] observed a characteristic feature where reef slopes were dominated by Calcarinamayori,C. spengleri,Heterostegina depressa,Amphistegina lessonii and Amphisteginaradiata, where, Amphisorushemprichii was said to be most available in less algal growth zone in the reef slopes. Neorotaliacalcar and Neorotaliagaimardi also occur in reef slopes with limited amount of interstitial space in between the coral rubbles which may be due to transport of carbonate sediments from the overlying reef flats.
Amphisorus hemprichii are best represented in sediments associated with seagrass. This has observed in the samples of Snob Island and Boat Island, where patches of seagrass observed. In addition, Renema et al., [43], recognized that the near shore is often dominated by Neorotaliagaimardi, and Marginoporavertebralis for high energy condition, support the present environment condition of the studied environment of these islands. Further, the genus Heterostegina, Amphistegina,Calcarina and Amphisorusalso support the higher energy settings of these locations. The less presence of eurytopic taxa such as Heterosteginadepressa and Amphisteginalessonii suggested that these locations are sandy and rock crevices are less in number [6]. In terrestrial influenced reefs, the reef flat is either dominated by Amphisteginalobifera or various Calcarinaspecies. Further, the above inferences are supported by the Renema’s [17], observation that the genus Amphisteginadominated regions are often associated with low algal abundance and moderate coral reef cover, whereas the calcarinids proliferate in algal dominated environments. Neorotaliagaimardi has also reported from algal environment from Kepulam Seribu, near Java [7]. Thus, Hottinger [68], Reiss and Hottinger [15], Hallock [52], and Renema and Troelstra [45] were suggested that, location of a reef (reef flat, reef slope, reef base and inter-reef) have an important role to play as parameters determining the LBF assemblage composition. However, this may not support this study, as the Andaman and Nicobar Island has a fringing reef only, i.e. terrace model coral reef, pertaining to limitations in studying with the above stated areas and distribution in the coral reef region.
From their study, Narayan and Pandolfi [69], stated that, substrate difference and depth preference among species [6] [7] [16] influenced their assemblage of individual taxa. Along with depth variation, the light availability, tolerance to terrestrial sediments even the occurrence of certain LBF species differed drastically. Renema [30], observed that species like Marginoporavertebralis, Parasoritesorbitolitoides and Alveolinellaquoyi are mostly found in inter reef region, i.e., between 15 m - 40 m depth was evinced by Jolly Buoy, Snob, Hobday and Grub Islands sites, where the depth goes around 20 m than the remaining islands environmental depth (15 m). Marginoporavertebralis also accompanied by other species of LBF assemblages belonging to genus Operculinaand Amphistegina. Amphisteginalobifera that is basically shallow living LBF species around 10 m [5] [6] [16] [47], but at deeper depth it is replaced by Amphisteginalessonii [45]. Basically, both Amphistegina species prefer hard, coral rubble or may be a loose coarse sandy substratum but over time, if algal growth takes place on the rocky sub-stratum, then Calcarinid species are found in abundance [6] [16] [17] [42]. Even Amphisteginabicirculata is well documented as deep dweller along with A. lessoniiwith depth range of about 40 m - 140 m [16] [42] [43] preferring firm substratum [44] or coarse sand [42] [43]. However, the present environment has less depth and algal mat has present, the above two species are very much less in this environment. As documented by Renema, [6], Amphisteginaradiata is found living in abundance from 10 m - 90 m depth. Highest abundance was recorded from 20 m depth from Okinawa, Japan by Hohenegger et al., [48]. They prefer carbonate rich sandy substrate with more tolerance to terrestrial influence or coral rubble as firm substrate to cling on the sides and inhabit in between the interstitial space [6] [15] [16] [44] [45]. However, the present study proved that the less abundance of this species due to the shallow water environment with less coral rubble. Calcarinaspengleri is well documented from a coral rubble with coralline algal growth or algal turf and Halimeda as substrate [6] [7] [45] with assemblage within 15 - 25 m depth. While Calcarinadefrancei exist within 3 - 25 m [6] [16] [42] [48], living inbetween interstitial gap of coral rubble covered with sand. Calcarinahispida are abundant at the base of filamentous algal mat [6] [50] [51] and may be found within >5 m depth, however, Hallock [23] [52] described its depth range to be from 10 m - 20 m from a strong currents region. Calcarinacalcarinoides were reported from firm substrate [50], epiphytically attached on algal turf from South China Sea [49]. Though its abundance is reported from <5 m, but it is mostly found down to 30 m water depth on the reef [48]. Neorotaliacalcar are epiphytically present on macro algae or sea grass (Halophila) on sand cay occuring within 10 m - 20 m in most places [7] [15] [45] [51]. Neorotaliagaimardi prefers depth within 30 m with algal turf surrounding or macro algae like Turbinaria and Sargassum [7] [17]. The above inferences clearly supported the present study environment has coral rubble with algal turf and current laden environment.
Diversity of larger benthic foraminifera (LBF) is said to decline to the east in the Pacific ocean and west of Indian Ocean and reef slopes are less differentiated by depth [16] [70]. Renema [30] studied and reported that terrestrial influence is one of the key driving factor for LBF assemblage composition that was not predicted before. According to Renema [30], Hottinger [19], and Hohenegger et al., [48], it has been suggested that depth distribution of LBF taxa is determined by the intensity of light and wavelength required by their algal symbiont.
Heterostegina depressa need broad range of light intensity (2%to 70% surface illumination), and its thick tests protects them from against irradiation, a cryptic life mode near the surface. Test construction enables life under strong hydrodynamic regimes. This species lives firmly attached to hard substrates, which help them to counteract against transportation by water movement. Nummulites venosus differs from Heterostegina depressa, having undivided chambers and its exclusive preference for coarse sand. It avoids high sediment movement with distribution range of 10 - 85 m depth [48] [53] [54]. N. venosus morphology shows distinct habitat preference with upper light intensity requirement limiting to that of Operculina ammonoides (80%) and lower limit of 2.5% surface illumination. H. depressa has wider depth distribution, 15 m - 40 m depth range [30] with larger tolerance to light and found in coral rubbles along with coralline algae [6] [7] [16] [45]. Heterostegina depressa and Nummulites venosus have less in abundance suggested that the present environment has very less rocky out crop as well comparatively less hydrodynamic conditions in these study areas.
LBF species like Soritesorbiculus, Amphisorushemprichii and Marginoporavertibralis share similar morphology but differ in their external features like thickness of their test, number of chamberlets and their forms and distribution of aperture on their peripheral region and the endosymbiont they have [66] [71]. Peneroplidae family is observed to host rhodophyte along with chlorophyte [62] [74] [75]. Parasoritesorbitolitoides and Amphisorushemprichii hosts dinoflagellates as well as chlorophytes [34] [40] and Soritesorbiculus is known to host dinoflagellate as their endosymbiont [71] while Marginoporavertebralis has red cyanobacteria along with dinoflagellate [76]. These are all porcelaneous wall structure which is said to be sensitive to much depth ranges or any changes in their surrounding environment. According to Lee [59], Bourne et al., [72] and Lee and Anderson, [4], it is observed that milioliida order mostly host chlorophyta, rhodophyta and dinoflagellates either at one point of time or may be one at a time depending upon the changes and adaptation they go through. Lee and Anderson [4] suggested that flexibility in the acceptance of the potential foraminiferal endosymbionts has considered favorable in chances of adaptation to broader range of environmental parameters. Several other experimental studies also demonstrate that algal symbiosis enhances calcification that contributes to the excellent growth of the foraminiferal test [38].
High specificity in their association with diatom or dinoflagellate is probably driven by selective recognition mechanisms of symbiont and biogeographical isolations [73]. Photosynthetic activity of endosymbionts is well known to provide foraminiferal host with the needed energy for survival and growth in any environment [24]. Symbiotic association proves to be necessary for the successful adaptation of Soritids to oligotrophic environment. In addition, Soritids are particularly abundant in the Indo-Pacific as they play very important role in biogeochemical mineral cycle.
The statistical plot for cluster analysis and multidimensional scaling (MDS) suggested that (Figure 2 and Figure 3) among these island environments, exhibited
![]()
Figure 3. Multi dimensional scaling (MDS).
![]()
Figure 4. Principal component analysis (PCA).
a maximum similar in their conditions. However, it may vary in the environment such as Grab Island as well as Jolly Buoy Islands, where the change of depth and its slope has an influence of their distribution was evinced from these figures (A & B). The principal component analysis (Figure 4) suggested that among the differences of environment in the inter islands will match with the intra islands environment, which again suggested that all these study area have almost similarity in their environmental conditions.
4. Conclusion
This present study from Mahatma Gandhi Marine National Park, Wandoor, Port Blair suggested that the stations selected for this study has a challenging environment with varying factors involved. The presence of few dominant LBF species, Calcarinacalcarinoides,C. spengleri,C. defrancei,Neorotalia calcar,N. gaimardi,Peneroplis pertusus,Marginopora vertebralis andSorites orbiculus suggested the availability of good fringing coral cover with commendable macro algae along with sea grass coverage, as they prefer such substratum for their epiphytic association. Even other LBF species Alveolinellaquoyi,Borelis schlumbergeri,Amphistegina lessonii,A. lobifera and Calcarinahispida,Nummulites venosus and Operculinaammonoides showed significant presence, indicating presence of sandy—carboniferous substrate with exposure to greater hydrodynamic energy. Their robustness is evidence to their adaptation to such rough environment. Nevertheless, presence of few more LBF species was observed, i.e., Amphisteginaradiata,A. papillosa,A. bicirculata andHeterostegina depressa that were in much negligible number and their indication suggested that the present studied environment need for still larger amount of coral rubble, high hydrodynamic and high sea grass presence, than what it has present now.
Acknowledgements
The authors want to thank The Head of the Department, Department of Ocean Studies and Marine Biology, Pondicherry University, Port Blair for providing all the infrastructure and basic facility needed for the study. Much grateful to the Dr. C. Sivaperuman, the Officer In Charge, Zoological Survey of India, Port Blair and his team for their assistance during the field work and sample collection.