Molecular Modeling and Antimicrobial Screening Studies on Some 3-Aminopyridine Transition Metal Complexes ()
1. Introduction
Pyridine derivatives are very important chemicals with many biological applications. They are known to have moderate-excellent activities towards variety of biological targets varying from microbial diseases to viral organisms and cancerous cells by interacting with enzymes, proteins and DNA [1] [2] [3] [4]. Oxime and hydrazone derivatives of pyridine exhibit high activity against influenza B-Mass virus, HIV and are also used as antidotes against poisoning by organophosphorus compounds [5] [6]. Many metal complexes with pyridine based ligands exhibit high cytotoxicity and have proved to be antitumor agents [7] - [11]. Molecular modeling (MM) is one of the fastest growing fields in science. It may vary from building and visualizing simple molecules in three dimensions (3D) to performing complex computer simulations on large proteins and nanostructures [12]. It is a collection of computer-based techniques for driving, representing and manipulating the structures and reactions of molecules depending on 3D structures. The techniques cover several issues among them computational chemistry, drug design, computational biology, nanostructures, and material science [13]. In recent years, density functional theory (DFT) has become an increasingly useful tool for theoretical studies. The success of DFT is due it is computationally less demanding than wave function based methods with inclusion of electron correlation [14]. In the present work seven Mn2+, Co2+, Ni2+, Cu2+ and Zn2+-3-aminopyridine (3-APy) complexes with variable stoichiometric ratios were prepared, characterized and their antibacterial activities were studied. Some complexes were tested for their in-vitro cytotoxic activity in human lung cancer cell lines (A-549 cell line), and structure-activity relationships were established. Also, the molecular structure of some selected metal complexes in view of chemical reactivity values such as chemical hardness, chemical potential electronegativity, electrophilicity index and HOMO-LOMO energy gap obtained theoretically were described.
2. Experimental
All reagents used were of the highest quality chemicals and were used without further purification. Aqueous solution of 1.00 mmol of metal salts was added with stirring to an ethanolic solution of 3-aminopyridine ligand with the desired molar ratio. The mixture was refluxed for ≈6 hours then cooled to room temperature, and the solid complexes so formed were filtered off and washed with distilled water followed by ethanol and dried under vacuum. Elemental analysis, spectroscopic studies and cytotoxic activity are as reported in our previous work [15].
2.1. Antimicrobial Screening
The antibacterial activity of some complexes toward some bacterial strains and fungi was evaluated by agar well diffusion method [16] [17]. The activity was evaluated by measuring the diameter of zone of inhibition in mm. A medium with DMF as solvent was used as a negative control whereas media with Ampicillin were screened separately for its standard antibacterial activity.
2.2. Molecular Modeling
The cluster calculations using DMOL3 program [18] in materials studio package [19] which is designed for the realization of large scale density functional theory calculation (DFT) were performed. DFT semi-core pseudopods calculations (dspp) were performed with the double numerica basis sets plus polarization functional (DNP). The DNP basis sets are of comparable quality to 6 - 31 G Gaussian basis sets [20].
3. Results and Discussion
3.1. Characterization of the Metal Complexes
The as-prepared complexes were characterized by different techniques and are proved to have the following structural formulae:
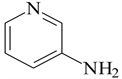
3-aminopyridine (3-APy)
1) MnBr2 ← 3-APy (1:6), C30H36N12MnBr2, M. Wt. 777.09, (% H 4.67, % C 46.33, % N 21.62 and % Mn 7.07)
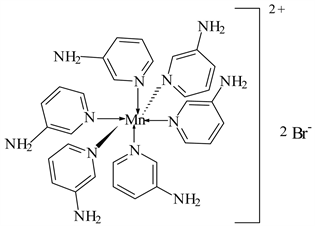
2) NiCl2 ← 3-APy (1:6), C30H36N12NiCl2, M. Wt. 692.19, (% H 5.24, % C 52.01, % N 24.28 and % Ni 8.37)
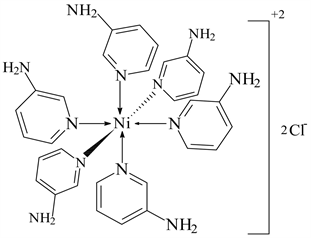
3) CoCl2 ← 3-APy (1:6), C30H36N12CoCl2 M. Wt. 694.54, (% H 5.22, % C 51.88, % N 24.20 and % Co 8.49)
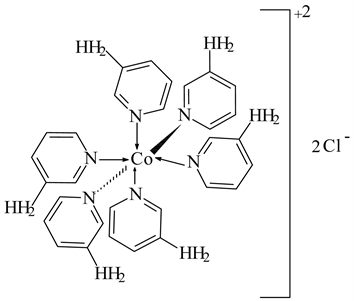
4) CuCl2 ← 3-APy (1:2), C10H16N4O2Cl2Cu, M. Wt. 357.00, (% H 4.52, % C 33.61, % N 15.69 and % Cu 17.63)
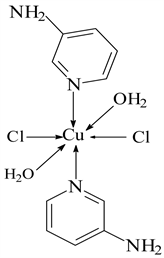
5) CuCl2 ← 3-APy (1:6), C30H36N12CuCl2, M. Wt. 697.19, (% H 5.19, % C 51.54, % N 24.04 and % Cu 9.09)
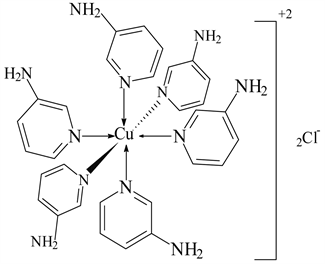
6) Cu(CH3COO)2 ← 3-APy (1:2), C14H22N4O6Cu, M. Wt. 405.08, (% H 5.47, % C 41.47, % N 13.83, % O 23.69 and % Cu 15.53)
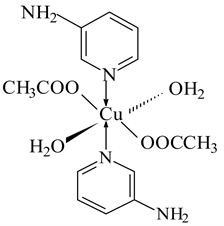
7) ZnCl2 ← 3-APy (1:2), C10H16N4O2Cl2Zn, M. Wt. 360.55, (% H 4.50, % C 33.52, % N 15.65, % Zn 17.86)
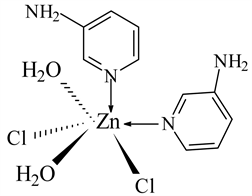
3.2. Thermal Analysis
Up to 800˚C, the prepared metal complexes degrade thermally through, more or less, three main steps. Representative thermogram is shown in Figure 1 and the thermal degradation patterns show that the physically adsorbed and coordinated water molecules are dehydrated from the coordination sphere in two successive steps within the temperature range 80˚C - 220˚C followed by full thermal decomposition at temperature higher than 335˚C leading to metal oxides as final products.
3.3. Infrared Spectra
The IR spectra of the complexes M ← 3-aminopyridine were studied in terms of their molecular structure. The most important band frequencies (cm−1) are cited in Table 1 from which it is found that the spectra exhibit broad band within the range 3400 - 3448 cm−1 and weak band within the range 2955 - 3024 cm−1 due to the stretching vibrations of OH and NH2 (VOH and VNH2) groups, respectively. This remarkable broadness and shifting to lower frequencies indicates their contribution in coordination process. This is supported by the appearance of new broad bands within the range 3400 - 3350 cm−1 and 574 - 545 cm−1 due to the stretching vibrations of coordinated water molecules and the M-O bond, respectively. The stretching vibration of the C=N group (VC=N) in the 3-aminopyridine moiety appears at 1545 - 1655 cm−1 range while that of the stretching vibration of the C-N group (VC-N) appears at 1516 - 1575 cm−1 range. The OH in-plane
![]()
Figure 1.Thermogram of the complex NiCl2 ← 3-APy (1:6).
![]()
Table 1. IR vibrational frequencies (cm−1) of some function groups of Mn2+, Co2+, Ni2+, Cu2+ and Zn2+ complexes with 3-aminopyridine.
deformation of the coordinated water molecules gives rise to band within the range 1018 - 1110 cm−1. The out-of-plane deformation of the OH group of the complexes is represented by the strong band within the range 852 - 740 cm−1, while the far infrared region of the spectra of the metal chelates shows new sets of bands within the region 525 - 573 and 483 - 527 cm−1 which are due to M-Cl and M-N bonds, respectively.
3.4. Electron Spin Resonance (ESR) Spectra
The X-Band ESR spectra of the powder CuCl2 ← 3-APy (1:2) and Cu(Ac)2 ← 3-APy (1:2) complexes were recorded at room temperature using DPPH as reference standard (c.f. Figure 2). The ESR exhibits anisotropic spectra with g⊥ > gII > ge (g e = 2.0036 free ion value) characteristic for compressed axial symmetry of the coordination sphere (one coordination axis (z) is significantly shorter than the other two (x, y) with dx2-y2 ground state. One unpaired electron in Cu (II) complex with 2B1g as ground state lies in dz2 orbital. Analysis of spectra gave the gII and g┴ values as cited in Table 2.
![]()
Table 2. ESR spectral parameters for Cu(II) complexes.
![]()
Figure 2.ESR spectra of Cu(CH3COO)2 ← 3-APy (a) and CuCl2 ← 3-APy (b).
3.5. Magnetic Moments and Electronic Absorption Spectra
Magnetic moments and electronic spectral data give information about the oxidation state and stereochemistry of the central metal ion in coordination complexes. The data are presented in Table 3.
The magnetic moment of Mn(II) complex is 5.85 BM indicating d5 high spin (t2g3 eg2) configuration,(spin only value (5.92 BM) while that for Co(II) complex is 5.17 BM indicating d7 high spin (t2g5 eg2) configuration (spin only value 3.88) which agree with octahedral range 4.3 - 5.2 BM. Ni(II) complexes showed the magnetic moment values of 3.20 BM consistent with their d8 (t2g6 eg2) octahedral environment. Cu(II) complexes showed magnetic moments of 1.77 BM, slightly higher than the spin-only value (1.73 BM) expected for one unpaired electron d9 (t2g6 eg3), offering the possibility of octahedral geometry [21]. These geometries are further confirmed by electronic transitions. The Zn (II) complex is observed to be diamagnetic as expected from its electronic configuration. The electronic spectral data (measured using Nujol mull technique) are used for assigning the stereochemistry of metal ions in the complexes based on the d-d transitions observed (c.f. Table 3).
3.6. Biological Activity
The antimicrobial and antifungal activities of selected complexes were tested against two bacterial; Staph. aureus, E. coli and two fungi; Asper. ocheratious, Asper. niger species. Standard drug; Ampicillin and DMF solvent control were screened separately for their antibacterial activity. The results and percent activity (c.f. Table 4) show that:
1) For antimicrobial activity, the tested complexes show higher activity towards Staph. aureus than that for E. coli. In the former case, MnBr2 (1:6) has the highest activity followed by CuCl2 (1:6), CuSO4 (1:2), CuCl2 (1:2), ZnCl2 (1:2) and NiCl2 (1:6) while CoCl2 (1:6) has no activity. For E. coli; the activity lies in the order CuCl2 (1:2) > CuCl2 (1:6) > ZnCl2 (1:2) > MnBr2 (1:6) > CoCl2 (1:6) > NiCl2 (1:6) > CuSO4 (1:2).
![]()
Table 3. Magnetic moments and spectral data for selected complexes.
![]()
Table 4. Antibacterial and antifungal activities of some complexes in terms of inhibition zone diameter (mm) and % activity index (% Ac. Ind.).
2) For antifungal activities, the tested complexes have very high activity towards Asper. ocheratious compared to that for Asper. niger where it was found that for Asper. ocheratious CoCl2 (1:6) and Cu(Ac)2 (1:2) have higher activity than ampicillin and other complexes showed very high activities. On the other hand, the tested complexes showed nearly no activities towards Asper. niger except for the complexes CuCl2 (1:2 and 1:6), Cu(Ac)2 (1:2) and ZnCl2 (1:2).
Inspection of the data cited above shows that, as a general trend, the activity of the complexes is higher than that of the free ligand. The increase in activity is simply due to the fact that chelation process reduces the polarity of the ligand due to partial sharing of its negative charge with metal ion favoring transportation of the complexes across the lipid layer of the cell membrane [22] [23]. This is in accordance with the calculated quantum chemical analysis data, gathered from molecular modeling studies (as will be shown later in Section 3.2.1), where it is found that complexes show a decrease in the HOMO-LOMO energy gap (ΔE) and increase in each of electronegativity (χ), chemical potential (µ) and electrophilicity (ω). This result suggests the very diffusion of the complexes into the bacterial membrane favoring the antimicrobial activity.
3.7. Antitumor Activity
The cytotoxic activities of some selected complexes were tested against Lung carcinoma cells A-549 cell line and compared to that of Vinblastine as a standard drug. The 50% inhibitory concentration (IC50) was estimated from graphic plots of the relation between surviving cells and complex concentration. The lethal concentrations (IC50) values with a brief comment on the data are listed in Table 5.
![]()
Table 5. The lethal concentrations (IC50) values and brief comment on the data of the complexes against Lung carcinoma under the experimental conditions.
From the data cited in the Table, it is clear that Co(II) and Cu(II) complexes have high activity toward lung carcinoma cell with a low IC50 value (81.6 ± 2.4 and 108. ± 2.7 µg/ml, respectively) while Zn(II) complex showed moderate activity. On the other hand, Ni(II) complex showed weak activity against the tested cell line (IC50 value 354 ± 8.7 µg/ml).
3.8. Molecular Orbital Calculations
3.8.1. Molecular Modeling
The molecular modeling, the total density function, deformation density function and frontier orbital energy (the HOMOs and LUMOs) for the ligand 3-aminopyridine and its Ni2+, Cu2+ and Zn2+ complexes were determined using DFT method. The quantum chemical parameters of the studied complexes were calculated as given in Table 6.
The HOMOs and LUMOs are known as Frontier molecular orbitals (FMOs), which play an important role for evaluating molecular chemical stability, and hardness-softness of the molecule [24]. The energy gap, ΔE (EHOMO − ELOMO), represents the chemical reactivity of compounds, (system of lower value of ΔE is more reactive). As depicted in Table 6, the free ligand (3-APy) has the largest energy gap (2.889 eV) which decreases in case of complex species indicating their stability. Another global reactivity descriptor electrophilicity index (ω) which describes the electron accepting ability of the systems quite similar to hardness and chemical potential. High values of electrophilicity index increases electron accepting abilities of the molecules. The latter values increase in case of complex species indicating the increase in their electron accepting abilities. The direction of the charge transfer is completely determined by the electronic chemical potential (μ) of the molecule because an electrophile is a chemical species capable of accepting electrons from the environment and its energy must decrease upon accepting electronic charge. Electronegativity (χ), chemical potential (µ), global hardness (η), global softness (σ), additional electronic charge (ΔNmax) and global electrophilicity index (ω) [25] [26] were calculated and listed in Table 6:
,
,
,
,
Some energetic properties of ligand 3-APy and its complexes were calculated by DFT method and are given in Table 7.
![]()
Table 6. The quantum chemical parameters of the studied complexes of ligand 3-APy.
![]()
Table 7. Some energetic properties of ligand 3-APy and its complexes calculated by DFT-method.
3.8.2. Geometry Optimization with DFT Method
The molecular structures, atomic numbering, representative bond angles along with the total density of the studied complexes are shown in Structures 1-4. The total density and spin density of the complex are shown in Figures 3-10. From the data given, it is clearly found that the bond angles of the free ligand are altered somewhat upon coordination.
1) The complex NiCl2: 3-APy (1:6)
![]()
![]()
Structure 1. Structure and selected bond angles of the complex NiCl2: 3-APy (1:6).
![]()
![]()
Figure 3.Total density and spin density using DFT method of the complex Ni: 3-APy (1:6).
![]()
![]()
Figure 4.Deformation density and 3D plots frontier orbital energies using DFT method for the complex Ni: 3-APy (1:6).
2) The complex CuCl2: 3-APy (1:2)
![]()
![]()
Structure 2. Molecular modeling, structure and selected bond angles of the complex CuCl2: 3-APy (1:2).
![]()
![]()
Figure 5.Total density and spin density using DFT method of the complex CuCl2: 3-3-APy (1:2).
![]()
![]()
Figure 6.Deformation density and 3D plots frontier orbital energies using DFT method for the complex CuCl2: 3-3-APy (1:2).
3) The complex Cu(Ac)2: 3-APy (1:2)
![]()
![]()
Structure 3. Molecular modeling, structure and selected bond angles of the complex Cu(Ac)2: 3-APy (1:2).
![]()
![]()
Figure 7.Total density and spin density using DFT method for the complex Cu(Ac)2: 3-APy (1:2).
![]()
![]()
Figure 8.Deformation density using and 3D plots frontier orbital energies using DFT method for The complex Cu(Ac)2: 3-APy (1:2).
4) The complex ZnCl2: 3-APy (1:2)
![]()
![]()
Structure 4. Molecular modeling, structure and selected bond angles of the complex ZnCl2: 3-APy (1:2).
![]()
![]()
Figure 9.Total density and deformation density using DFT method the complex ZnCl2 ← 3-APy (1:2).
![]()
Figure 10.3D plots frontier orbital energies of the complex ZnCl2 ← 3-APy (1:2) using DFT method.
4. Conclusion
Seven Mn2+, Co2+, Ni2+, Cu2+ and Zn2+-3-aminopyridine (3-APy) complexes with variable stoichiometric ratios were prepared, characterized then, their antibacterial and cytotoxic activities toward some bacteria, fungi and human lung cancer were studied. The molecular structure of some selected metal complexes in view of chemical reactivity values such as chemical hardness, chemical potential electronegativity, electrophilicity index and HOMO-LUMO energy gap obtained theoretically were described. The latter data are used to understand their biological activities. The small HOMO-LUMO gap value and the chemical reactivity values show that the complexes are biologically active. It is worthy to mention that the experimental results belong to solid phase and theoretical calculations belong to gaseous phase. In the solid state, the existence of the crystal field along with the intermolecular interactions have connected the molecules together, which result in the differences of bond parameters between the calculated and experimental values.