Using an Absolute Cavity Pyrgeometer to Validate the Calibration of a Secondary Standard Pyrgeometer Outdoors, Independent from the Reference Value of the Atmospheric Longwave Irradiance ()
1. Introduction
The pyrgeometer model PIR was installed outdoors on an aluminum plate that was connected to a temperature controller, see Photo 1. Adjusting the temperature controller to decrease the pyrgeometer’s body temperature changed the pyrgeometer’s thermopile output. If the incoming radiation was stable, then the slope of the change in the pyrgeometer’s output irradiance (Wout) versus the change in the thermopile output voltage (V) would equal the pyrgeometer outdoors responsivity (RS), independent from the absolute value of the atmospheric longwave irradiance [1]. We then evaluated the results of the method using two Absolute Cavity Pyrgeometers (ACPs), ACP95F3 and ACP10F3. The ACPs were installed on temperature controllers and operated as described in [2]. The described method addressed the inherent problems related to the spectral difference between the blackbodies and the atmospheric irradiance by calculating RS from the clear sky outdoor calibration and using the actual atmospheric irradiance as the calibration source.
Section 2 describes the procedure, equations, and the mathematical representation of the rate of change of V versus Wout.
Section 3 shows the procedure results and the outdoor evaluation of this procedure. The evaluation compared the measured irradiance by the PIR against the average irradiance measured by ACP95F3 and ACP10F3.
Section 4 is the conclusion.
2. Procedure
The ACPs and PIR were deployed outdoors from July 20 to August 19, 2020. Two equations were used to calculate the atmospheric longwave irradiance measured by the PIR, as described below in NREL and PMOD equations.
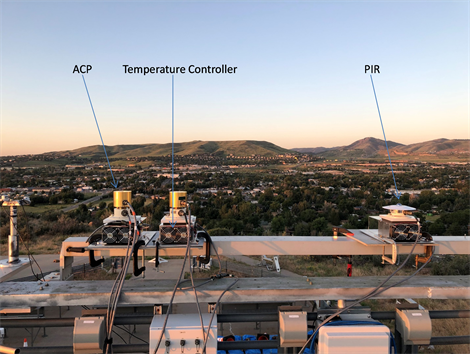
Photo 1. Outdoor set up from left to right of ACP95F3, ACP10F3, and PIR on top of temperature controllers.
NREL Equation [3]
(1)
where:
Watm is the atmospheric longwave radiation in W/m2.
K0, K2, and K3 are the calibration coefficients of the pyrgeometer, calibrated at the PMOD [4].
K1 is the reciprocal of the PIR’s RS, calculated from the outdoor calibration described below.
V is the pyrgeometer thermopile output, in microvolts.
Wr is the pyrgeometer receiver radiation =
, and
,
where:
σ Stefan-Boltzman constant = 5.6704 × 10−8 W/m2/K4.
Tc pyrgeometer case temperature, in Kelvin.
S Seebeck coefficient = 39 V/K.
n number of thermopile junctions = 56 junctions.
E thermopile efficiency factor = 0.65 (manufacturer specification).
K4 thermopile efficiency factor equal to
= 0.0007044 K·uV−1.
Wd is the pyrgeometer dome radiation =
, where Td is the dome temperature in Kelvin.
Equation (1) is rewritten in the following form:
(2)
where:
Wnet is the net irradiance measured by the pyrgeometer thermopile.
Wout is the outgoing irradiance from the pyrgeometer.
(3)
A fundamental principle for this calibration procedure is to lower the outgoing irradiance while the atmospheric longwave irradiance (Watm) is constant, i.e., stable during clear sky conditions to within 1 W/m2 from the start to end of the calibration, at least 7 minutes. Lowering Wout was achieved by cooling the pyrgeometer’s case using the temperature controller. While lowering Wout, all signals from the pyrgeometer were measured every 10 seconds (i.e., thermopile output voltage, Td, and Tr). Differentiating Equation (2) with respect to time then yields:
(4)
If Watm is assumed constant, Equation 4 then yields:
(5)
Equation (5) implies that the change of Wout versus the change of V yields K1, which is independent from the absolute value of Watm.
Once K1 was calculated using the above procedure, Equation (1) was used to calculate the measured atmospheric longwave irradiance for 2 hours, and then the procedure was repeated from a solar zenith angle of >95˚ (PM) to <95˚ (AM).
PMOD Equation [4]
(6)
where C is the pyrgeometer responsivity and Tb is the case temperature.
As in the NREL method described above, where Watm is assumed to be constant, differentiating Equation 6 with respect to time yields:
(7)
For simplicity, Equation (7) is rewritten as:
(8)
where
and
.
Equation (8) implies that the change of F versus the change of Wout yields C, which is independent from the absolute value of Watm.
The ACP’s Measurement Equation [2]
To measure the atmospheric longwave irradiance:
(9)
where:
Watm is the atmospheric longwave irradiance (W/m2).
K1 is the reciprocal of the ACP’s responsivity (W/m2/uV).
V is the thermopile output voltage (uV).
ε is the gold emittance.
K2 is the emittance of the black receiver surface.
Wr is the receiver irradiance (W/m2).
Wc is the concentrator irradiance (W/m2).
τ is the ACP’s throughput.
3. Results
The measurement uncertainty was calculated using the following equation:
(10)
where U95ACP equals ±2 W/m2 with respect to SI [2], using the NREL equation U95PIR equals ±2.88 W/m2 with respect to ACP; therefore, U95PIR equals ±3.51 W/m2 with respect to SI. Using the PMOD equation, U95PIR equals ±2.22 W/m2 with respect to ACP; therefore, U95PIR equals ±2.99 W/m2 with respect to SI.
Photo 1 shows the outdoor set up of ACP95F3, ACP10F3, and PIR. Table 1 is a sample list of the calculated K1 for the PIR (NREL), ACP95F3, ACP10F3, and C for PIR (PMOD). Figure 1 and Figure 2 show Wnet versus V for ACP95F3 and ACP10F3. Figure 3 shows Wout versus V for the PIR using the NREL equation. Figure 4 shows Wout versus F for the PIR using the PMOD equation. Figure 5 shows the average irradiance of the ACPs and the PIR using NREL and PMOD equations. Figure 6 shows the difference between the ACPs average irradiance and the PIR irradiance using NREL and PMOD Equations. Figure 7 shows the atmospheric water vapor content.
![]()
Figure 1. Wnet versus thermopile output voltage during the calibration of ACP95F3.
![]()
Figure 2. Wnet versus thermopile output voltage during the calibration of ACP10F3.
![]()
Figure 3. Wout versus thermopile output voltage during the calibration of PIR31197F3 using NREL equation.
![]()
Figure 4. Wout versus F during the calibration of PIR31197F3 using PMOD equation.
![]()
Figure 5. ACPs average irradiance and PIR31197F3 irradiance versus MST.
![]()
Figure 6. Difference between ACP’s average irradiance and PIR31197F3 irradiance versus MST.
![]()
Figure 7. Water vapor content during data collection versus MST.
![]()
Table 1. Sample of the calculated K1 for PIR (NREL), ACP95F3, ACP10F3, and C for PIR (PMOD).
4. Conclusion
We conclude that using this procedure will result in calibration coefficients that are independent from the absolute value of the atmospheric longwave irradiance. Based on the results, it is possible to achieve an uncertainty of ±3.51 W/m2 using the NREL equation and an uncertainty of ±2.99 W/m2 using the PMOD equation with respect to SI. These results suggest that the PIR calibration method might be useful in addressing the international need for a secondary standard pyrgeometer traceable to SI.
Acknowledgements
The authors thank the Atmospheric Radiation Program, the NREL Metrology Laboratory, and the NREL Photovoltaic program for providing the funds for this article. The authors also thank Julian Gröbner of PMOD/WRC for calibrating the pyrgeometer used in this article and for pre-reviewing the article before submitting it to the Journal. We strongly thank Martina Stoddard for her persistent administrative support.